Dina S. Soliman1,3,4, Samah Kohla1,3,6, Shehab Fareed2, Susanna Akiki1,3, Aliaa Amer1, Ibrahim Ganwo1, Prem Chandra5, Halima El-Omri2 and Feryal Ibrahim1.
1 Department
of Laboratory Medicine and Pathology, National Centre for Cancer Care
and Research, Hamad Medical Corporation, Doha, Qatar.
2
Department of Hematology and Medical Oncology, National Centre for
Cancer Care and Research, Hamad Medical Corporation, Doha, Qatar.
3 Weill Cornell Medicine-Qatar, Doha, Qatar.
4 Department of Clinical Pathology, National Cancer Institute, Cairo, Egypt.
5 Medical Research Centre, Academic Health System, Hamad Medical Corporation, Doha, Qatar.
6 Clinical Pathology Department, Al-Azhar University, Cairo, Egypt.
Correspondence to:
Dina S. Soliman. Department of Laboratory Medicine and Pathology,
National Centre for Cancer Care and Research, Hamad Medical
Corporation, Doha, Qatar. E-mail:
DSoliman@hamad.qa
Published: September 1, 2022
Received: June 3, 2022
Accepted: August 12, 2022
Mediterr J Hematol Infect Dis 2022, 14(1): e2022067 DOI
10.4084/MJHID.2022.067
This is an Open Access article distributed
under the terms of the Creative Commons Attribution License
(https://creativecommons.org/licenses/by-nc/4.0),
which permits unrestricted use, distribution, and reproduction in any
medium, provided the original work is properly cited.
|
To the
editor
Sideroblastic
anemias (SA) is a group of inherited and acquired bone marrow (BM)
disorders defined by pathological iron accumulation in the mitochondria
of erythroid precursors.[1,2] SA pathogenesis
mechanisms can be primarily linked to impaired heme biosynthesis,
defect in Fe-S biogenesis pathways, and impaired synthesis of
mitochondrial and cytosolic proteins essential for heme synthesis.
According
to the International Working Group on Morphology of Myelodysplastic
Syndrome (IWGM-MDS); ring sideroblasts (RS) are defined as
erythroblasts in which there is a minimum of five siderotic granules
covering at least one-third of the circumference of the nucleus.[3] SA is a rare disease affecting fewer than 200,000 people in the US [4].
Due to the low incidence and prevalence and the heterogeneity of the
causative factors, no certain statistical data on the epidemiology of
this disorder.
The SA is traditionally classified into
congenital sideroblastic anemia (CSA) and acquired forms (ASA). ASA is
further subclassified into two groups, clonal neoplastic disorders and
benign disorders due to reversible metabolic factors.
Acquired
clonal SA comprises myeloid stem cell disorders associated with RS,
which was classified according to the 2016 revised World Health
Organization (WHO) Classification into three categories:
Myelodysplastic syndrome (MDS) with ring sideroblasts and
single-lineage dysplasia (MDS-RS-SLD), MDS with ring sideroblasts and
multilineage dysplasia (MDS-RS-MLD), and MDS/MPN with ring sideroblasts
and thrombocytosis (MDS/MPN-RS-T). As their names suggest, the
classification is based on detecting RS accompanied by dysplasia in one
or multiple hematopoietic lineages, or anemia, thrombocytosis, and
features of a myeloproliferative neoplasm.[2]
Conferring
to WHO criteria: MDS-RS-SLD, MDS-RS-MLD, and MDS/MPN-RS-T ring
sideroblasts should be >15% of the BM erythroid precursors; however,
if SF3B1 mutation is detected, the diagnosis can be made with >5% ring sideroblasts marrow erythroid precursors.[2] For other listed categories of myeloid neoplasms, no specific cut-off RS percentage is required.
Mutations
in the spliceosome that mediates the maturation of primary mRNA
transcripts to mature mRNAs lacking introns have been identified as
common in MDS-RS. Specifically, the acquired heterozygous somatic
mutations in SF3b1 are the strongest molecular correlate of MDS-RS. SF3B1 mutations are present in between 70% and 90% of MDS-RS and, in many, it is the single detectable clonal marker.[5]
Non-neoplastic
causes of RS include copper deficiency (which may be induced by a high
dose of zinc administration), alcohol, toxins, and drugs (e.g.,
isoniazid).[6] Unlike in MDS-RS, patients with CSA
tend to present at a much younger age and with microcytic (rather than
macrocytic) anaemia.[7]
The design of this study
is mixed (retrospective and prospective); we have analysed the
clinical, pathologic, and molecular data of 15 cases of ASA diagnosed
in Hamad Medical Corporate, in Qatar, between March 2015 and March
2022. The next generation sequence (NGS) panel designed to study
targeted regions in 30 genes recurrently mutated in myeloid neoplasia
(including SF3B1 mutations and TP53) was then performed (according to specimens' availability) and consequently analysed.
The study had ethical approval from IRB (Institutional research board; # MRC-04-22-209). Patients were waived from consent as it is a retrospective study.
ASA diagnosis was established by the presence of RS detected by Prussian blue staining (Perls' reaction) on BM aspirate smears (Figure 1).
All included cases were examined independently by two experienced
hematopathologists. Diagnosis and subclassification of ASA were made on
BM specimens according to WHO 2016 classification. Relevant clinical,
hematologic, and BM pathologic findings, including assessment of the
percentage of trilineage dysplasia (a cut-off of 10%), flow cytometry
immunophenotyping for myeloid neoplasms and cytogenetics data, were
analysed. NGS panel for myeloid neoplasia was performed (according to
specimens' availability) and consequently analysed. Clinical and
laboratory data, including the disease course and outcome, were also
retrieved and analyzed.
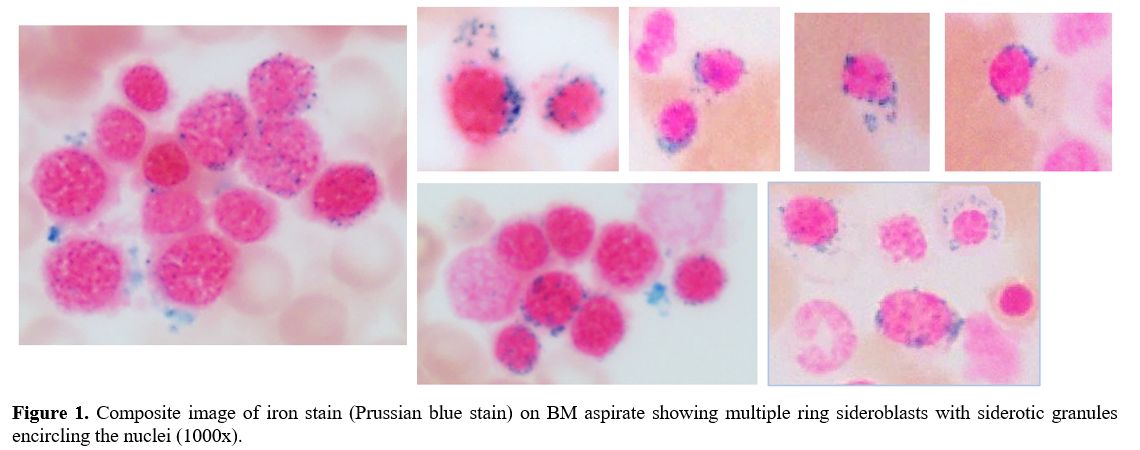 |
Figure 1. Composite image
of iron stain (Prussian blue stain) on BM aspirate showing multiple
ring sideroblasts with siderotic granules encircling the nuclei (1000x). |
Associations
between two or more qualitative variables across two independent groups
(clonal and non-clonal SA) were assessed using the Fisher Exact
Chi-square test or Pearson Chi-square as appropriate. Quantitative data
and outcomes measured across two groups were analysed using the
Mann-Whitney U test (due to skewed or non-normal data distribution).
All P values presented were two-tailed, and P values <0.05 were
considered statistically significant. All Statistical analyses were
performed using statistical packages SPSS version 27.0 (Armonk, NY: IBM
Corp) and Epi-info (Center for Disease Control and Prevention, Atlanta,
GA) software.
Fifteen patients of ASA were detected and
clustered into two main groups: clonal SA (associated with a
hematologic neoplasm) (10 cases, 66.7%) and SA secondary to non-clonal
causes (5 cases, 33.3%). The latter included: SA Secondary to copper
deficiency (two cases), SA secondary to pyridoxine deficiency, and the
last group included two patients where the exact cause of SA remained
unrevealed and classified accordingly as idiopathic SA (Tables 1 and 2).
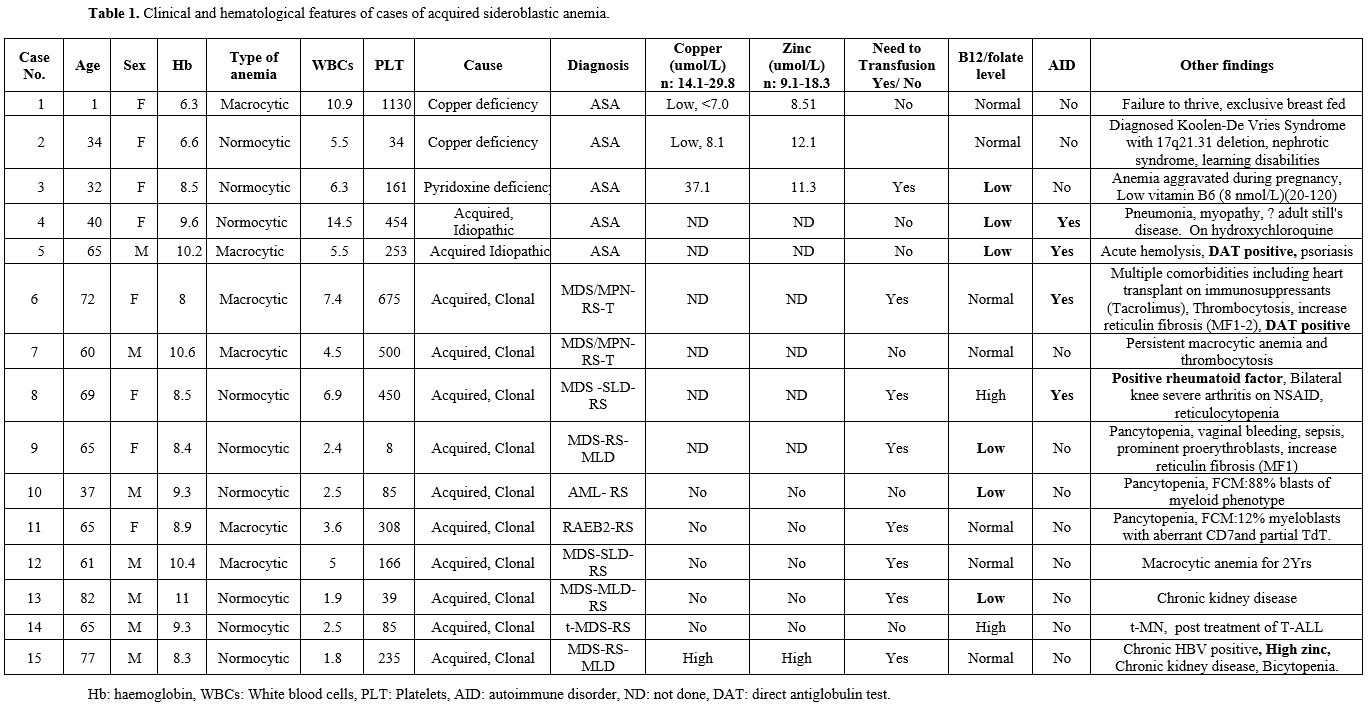 |
Table 1. Clinical and hematological features of cases of acquired sideroblastic anemia. |
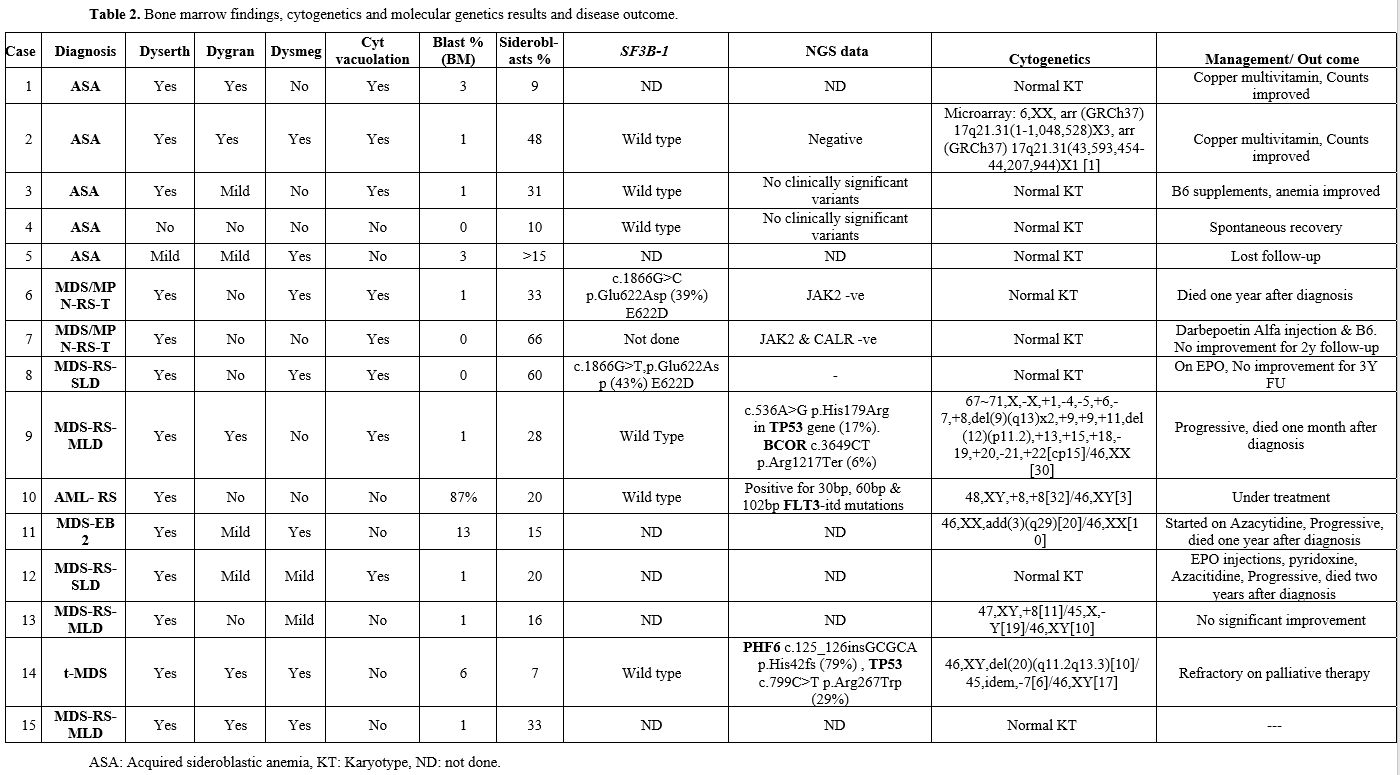 |
Table 2. Bone marrow findings, cytogenetics and molecular genetics results and disease outcome. |
ASA
in our cohort was diagnosed in all age groups, with a median age of 65
years (IQR 37- 69 years), and almost equally represented between male
and female gender. All patients had a significant degree of anemia; the
median hemoglobin was 8.5 g/dL (IQR 8-10.2), 6 cases were macrocytic,
and 8 were normocytic normochromic anemia. None of the cases in our
cohort showed microcytic hypochromic anemia. The median white blood
cell count (WBC) was 5×109 /L (IQR 2.5-6.9), and the median platelets count was 235 ×109 /L (IQR 39.0- 454).
Dyserythropoiesis
was the most striking finding (in 14/15 (93.3%), mostly represented by
cytoplasmic abnormalities: cytoplasmic irregularities/inclusions with
prominent cytoplasmic vacuolation in 9/15 cases (60%).
Dysmegakaryopoiesis was found in 9/15 patients (60%). Dysgranulopoiesis
was detected in 8/15 (53.3%).
RS were detected at variable
percentages ranging between 7-66% (median 20, IQR 13-33). Abnormal
karyotype (KT) was detected in 5/14 (35.7%) myeloid neoplasms with RS.
No cytogenetic abnormality was detected within the group of non-clonal
SA.
NGS was performed in 8 out of 15 cases, including 3/5 of the non-clonal cases. SF3B1 mutatin was found in 2/8 patines (25%), one with MDS-SLD-RS and the other with MDS/MPN-RS-T. In six cases, SF3B1 was wild type. Further genetic mutations detected by NGS included the TP53 gene (case 9 & case 14), PHF6, FLT3-itd, and BCOR mutations.
Clinically,
60% (9/15) of ASA in our cohort had significant anemia that required
regular blood transfusions. In addition, 6/15 (40%) cases had a
concurrent low serum B12/folate. Associated autoimmune disorders,
including DAT-positive autoimmune hemolytic anemia (two cases), adult
Still's disease, and autoimmune arthritis, were observed in 4/15
patients (26.6%).
Comparison between acquired clonal (neoplastic) cases and non-clonal SA (Table 3).
SA secondary to an associated myeloid neoplasm was predominant among
the elderly, with 8 out of 9 cases diagnosed at an age > 60. The
median age was 65 (IQR 60.75- 73.25 years), while non-clonal SA was
more represented within the younger age group, with a median age of 34
(16.5-52.5 years). This difference was statistically significant
(P=0.016). Interestingly, most non-clonal SA (80%) were female patients.
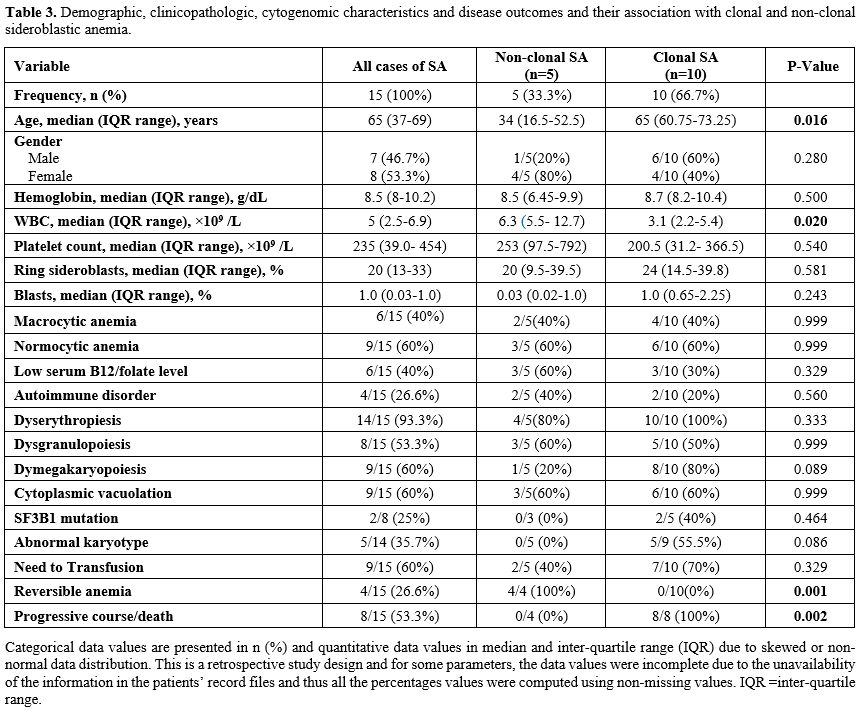 |
Table 3 Demographic,
clinicopathologic, cytogenomic characteristics and disease outcomes and
their association with clonal and non-clonal sideroblastic anemia. |
No
statistically significant difference (P>0.05) was observed between
the two groups regarding the haemoglobin, platelets count, or the type
of anemia. However, WBC was significantly higher in non-clonal SA
(median 6.3, IQR 5.5-12.7) compared to the clonal SA group (median 3.1,
IQR 2.2-5.4), P=0.020.
Dyserythropoiesis and dysgranulopoiesis
were equally present, with no significant morphologic difference
between both groups regarding the type of dyserythropoietic changes.
However, the cytoplasmic vacuolations were well defined and numerous in
non-clonal SA (Figure 2A), while in clonal SA, there was a focal clearing of the cytoplasm rather than definitive vacuoles (Figure 3B).
It is worth noting that the granulocytes in the group of non-clonal SA
(especially those secondary to copper or pyridoxine deficiency) showed
significant nuclear holes/well-defined defect/vacuolation within the
nuclear chromatin (Figure 2B),
in addition to the cytoplasmic vacuolations which were previously
described in cases of copper deficiency. The latter finding was not
appreciated within the MDS-associated SA, which showed abnormal
granulation &/abnormal segmentation (Figure 3A).
Dysmegakaryopoiesis (Figure 3C)
was most frequently detected among cases of clonal SA (8/10, 80%)
compared to non-clonal cases (1/5, 20%). However, this difference was
statistically insignificant (P=0.089). On the other hand, RS percentage
tends to be higher in clonal SA, with no statistically significant
difference (P=0.581).
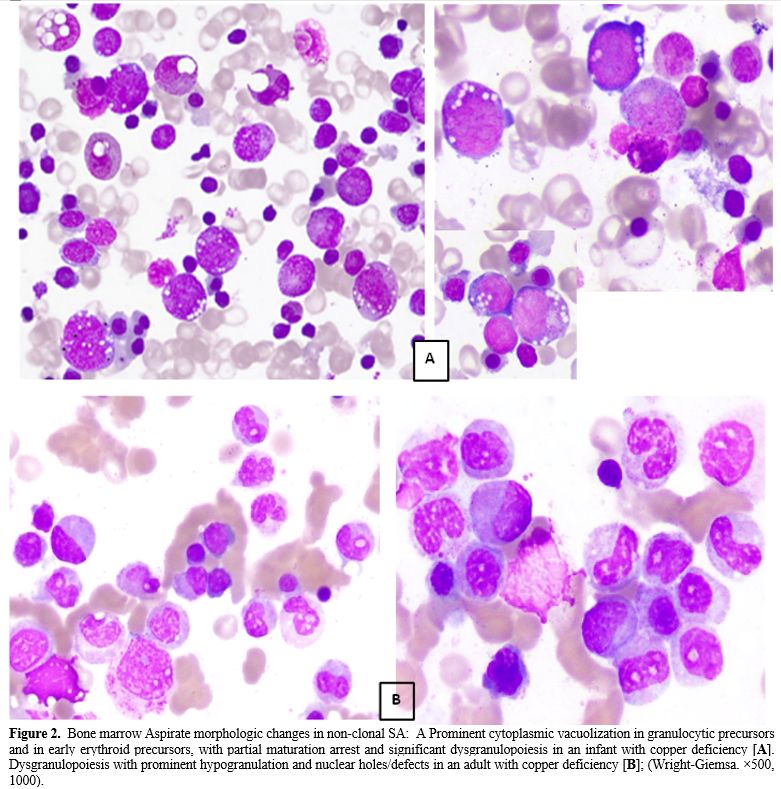 |
Figure 2. Bone marrow Aspirate morphologic changes in non-clonal SA: A
Prominent cytoplasmic vacuolization in granulocytic precursors and in
early erythroid precursors, with partial maturation arrest and
significant dysgranulopoiesis in an infant with copper deficiency [A]. Dysgranulopoiesis with prominent hypogranulation and nuclear holes/defects in an adult with copper deficiency [B]; (Wright-Giemsa. ×500, 1000). |
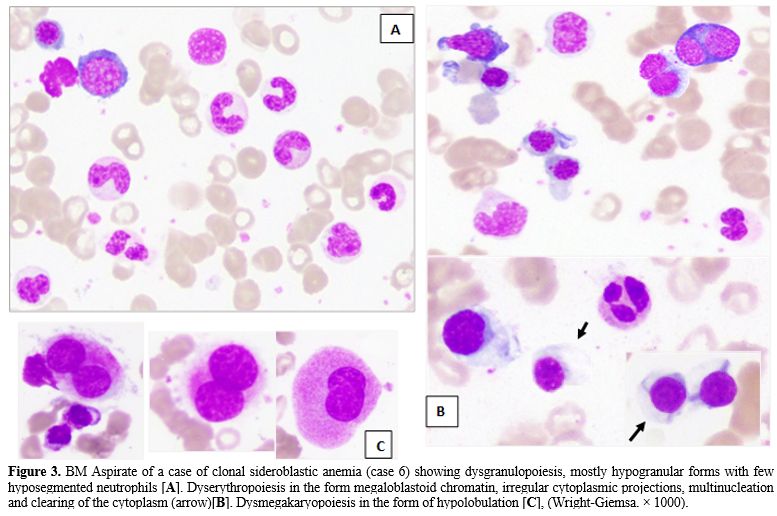 |
Figure 3. BM Aspirate of a
case of clonal sideroblastic anemia (case 6) showing dysgranulopoiesis,
mostly hypogranular forms with few hyposegmented neutrophils [A].
Dyserythropoiesis in the form megaloblastoid chromatin, irregular
cytoplasmic projections, multinucleation and clearing of the cytoplasm
(arrow)[B]. Dysmegakaryopoiesis in the form of hypolobulation [C], (Wright-Giemsa. × 1000). |
Clinically,
not much difference between both groups regarding the need for blood
transfusion. However, anemia was reversible in all cases with
non-clonal SA, while none of the subjects with clonal SA had
significant improvement (P=0.001). Vice versa, all of them with
clonal SA had progressive disease and fatal course (100% vs. 0%,
P=0.002). Due to the smaller number of cases, all comparative analyses
were performed above as an exploratory statistical analysis; therefore,
the derived statistical inferences might limit their conclusiveness and
generalizability.
Acquired copper deficiency is a
rare disorder associated with gastric bypass surgery or in cases with
total parenteral nutrition or exclusively breastfed babies (as seen in
case 1).[8]
In this patient, the prominent
cytoplasmic vacuolization was the key to the diagnosis, confirmed by
low serum copper. Furthermore, a positive therapeutic trial of copper
(in high concentration) led to a significant improvement of the
peripheral blood counts, which again dropped after copper supplements
had been stopped. Finally, FCM analysis on BM aspirate of this baby
showed an aberrant loss/down-regulation of CD33 on blasts,
granulocytes, and monocytes, which is a rare occurrence of uncertain
significance and might be a sign of dysmyelopoiesis.
In case
number 2, the patient was initially misdiagnosed as MDS-RS based on the
prominent multilineage dysplasia. However, no evidence of clonality was
detected, with normal KT, negative SF3B-1,
and no clinically significant variants identified using NGS.
Additionally, it was observed that the CBC parameters improved
significantly after hemodialysis. Copper serum level was then measured
and found to be extremely low; hence, SA secondary to copper deficiency
was concluded. Pancytopenia due to copper deficiency in a haemodialysis
patient has been previously reported by Melero et al.[9]
Furthermore, excessive zinc intake and copper deficiency induced
SA/pancytopenia during maintenance haemodialysis has also been reported
by Marumo A and his group.[10]
SA diagnosed
during pregnancy is even a rarer event. In Case 3, the anemia was
aggravated during pregnancies and normalized in between. This behaviour
had pointed to possible vitamin/trace element deficiency owed to
increase demand during pregnancy, especially since she had an
associated B12 defect. Although CSA could not be entirely ruled out in
this patient (as familial mutations were not done), however, the type
of anemia (normocytic rather than microcytic), a lacking family
history, absence of dysplasia, and detection of wild-type SF3B-1 gene, had all favoured an acquired cause.
Because
of the crucial role of pyridoxal phosphate (the active form of vitamin
B6) as an essential cofactor for ALAS2, a severe deficiency in vitamin
B6 due to alcohol intoxication, increased demands, malnutrition/
malabsorption could lead to SA.[11]
It is worth
noting that 4 out of 14 cases (28%) had an associated autoimmune
disorder with DAT-positive hemolytic anemia found in two patients.
In
cases (4 & 5), the exact cause of SA remained unrevealed, and there
was no evidence of clonality detected. Interestingly, both patients had
associated autoimmune disorder, with one patient having an adult
Still's disease on hydroxychloroquine (case 4); in the other patient,
SA was associated with DAT-positive autoimmune hemolytic anemia. We did
not find a reported association between hydroxychloroquine and SA.
This
association between SA and autoimmune disorders was rarely documented
in the literature; however, a transient appearance of RS in the acute
phase of secondary hemolytic anemia was reported by Wang and his group.[12]
Interestingly,
an associated megaloblastic anemia was found in 6/15 cases (40%) of
ASA; including 3 non-clonal cases and 3 cases associated with myeloid
neoplasms.
AML with RS is rarely encountered and can be seen in de
novo AML and secondary AML on top of MDS. We had a single case of a
male patient diagnosed at a relatively younger age (37 years old) and
considered the youngest among all groups of myeloid neoplasms with RS
in our cohort. Martin-Cabrera et al.[13] recently reported that AML with RS shows a unique molecular signature straddling secondary AML and de novo AML.
In our cohort, NGS (including SF3B-1
mutation) was performed in 8 out of 15 cases, including 3/5 of the
non-clonal cases. Within the group of clonal SA, clonality was
established in 7/10 cases either by abnormal KT and/or detection of
clinically significant variant using the NGS technique. Although
molecular testing could not be performed in the remaining three cases
(Case # 7, 12 and 15) due to lack of material, those cases had
convincing evidence of MDS based on the striking morphologic findings
and the aggressive disease course. TP53 mutation was detected in two
cases in our cohort (cases 9 and 14). According to a recent large-scale
genetic profiling study on MDS with RS, focusing on SF3B1-wild type
(WT) patients, the group found that in SF3B1-wt patients, the most
frequent mutation was TP53 at 61% (n = 92).[14]
The
treatment of SA relies on the underlying aetiology but remains
principally supportive with packed red cell transfusion for symptomatic
patients, vitamin B6 supplementation, and iron chelation therapy for
iron overload. Unlike SA due to myeloid neoplasms, the prognosis was
favourable in all cases of non-neoplastic SA (in our series), with
recovery of anemia either after replenishment of the deficient element
(copper/pyridoxine) or spontaneous recovery in cases with idiopathic SA.
In
conclusion, Although SA is uncommon and some forms are rare, it should
be considered in patients with unexplained persistent anemia of any
severity. It is sometimes difficult to distinguish congenital from
acquired causes or to reach the exact cause of acquired SA and
differentiate clonal from benign. The latter differentiation is crucial
since acquired SA may have significant myelodysplasia, which could not
be distinguished from MDS based solely on morphologic findings.
Compared to the non-clonal group, the neoplastic SA presents in the
elderly and tends to have lower WBC, higher blasts and RS, more
frequent dysmegakaryopoiesis, and a more aggressive clinical course
with fatal outcome.
Reaching a specific diagnosis requires a
multiparametric approach relying on multiple factors, including the age
of clinical onset, type of anemia (microcytic versus
normocytic/macrocytic), an associated syndromic/dysmorphic feature, the
degree of myelodysplasia, blasts percentage and most important the
detection of clonal cytogenetics/ molecular genetic marker.
References
- Bottomley SS, Fleming MD. Hematology/Oncology Clinics, 2014 https://hemonc.theclinics.com
- Arber
DA, Orazi A, Hasserjian R, et al. The 2016 revision to the World Health
Organization classification of myeloid neoplasms and acute leukemia.
Blood. 2016;127(20):2391-2405. https://doi.org/10.1182/blood-2016-03-643544 PMid:27069254
- Mufti
GJ, Bennett JM, Goasguen J, Bain BJ, Baumann I, Brunning R, et al.
Diagnosis and classification of myelodysplastic syndrome: International
Working Group on Morphology of myelodysplastic syndrome (IWGM-MDS)
consensus proposals for the definition and enumeration of myeloblasts
and ring sideroblasts. Haematologica. 2008;93(11):1712-7. https://doi.org/10.3324/haematol.13405 PMid:18838480
- Brissot
P, Bernard DG, Brissot E, Loréal O, Troadec MB. Rare anemias due to
genetic iron metabolism defects. Mutat Res Rev Mutat Res. 2018 Jul -
Sep; 777:52-63. https://doi.org/10.1016/j.mrrev.2018.06.003 PMid:30115430
- Papaemmanuil
E, Gazzola M, Boultwood J, et al. (2011). Somatic SF3B1 mutation in
myelodysplasia with ring sideroblasts. N Engl J Med. 365:1 384- 95. https://doi.org/10.1056/NEJMoa1103283 PMid:21995386 PMCid:PMC3322589
- Alcindor T, Bridges KR (2002). Sideroblastic anaemias. Br J Haematol. 116:733-43. https://doi.org/10.1046/j.0007-1048.2002.03378.x PMid:11886376
- Ohba
R, Furuyama K, Yoshida K, et al. Clinical and genetic characteristics
of congenital sideroblastic anemia: comparison with myelodysplastic
syndrome with ring sideroblast (MDS-RS). Ann Hematol. 2013. 92:1-9.
https://doi.org/10.1007/s00277-012-1564-5 PMid:22983749 PMCid:PMC3536986
- Wazir
SM, Ghobrial I. Copper deficiency, a new triad: anemia, leucopenia, and
myeloneuropathy. J Community Hosp Intern Med Perspect. 2017;7:265-8. https://doi.org/10.1080/20009666.2017.1351289 PMid:29046759 PMCid:PMC5637704
- López
Melero E, Ruíz-Roso G, Botella I, Ortego Pérez S, Delgado M, Fernández
Lucas M. Pancytopenia due to copper deficiency in a hemodialysis
patient. Nefrología.
- Marumo A, Yamamura
T, Mizuki T, Tanosaki S, Suzuki K. Copper deficiency induced
pancytopenia after taking an excessive amount of zinc formulation
during maintenance hemodialysis. J Res Med Sci
2021;26:42.2019;39:451-452. https://doi.org/10.4103/jrms.JRMS_25_19 PMid:34484374 PMCid:PMC8384003
- Konopka L, Hoffbrand AV. Haem synthesis in sideroblastic anaemia. Br J Haematol. 1979;42(1):73-83. https://doi.org/10.1111/j.1365-2141.1979.tb03699.x PMid:465361
- Fei
Wang, Geng Wang; Transient appearance of ring sideroblasts in
peripheral blood in the acute phase of secondary hemolytic anemia.
Blood 2019; 133 (9): 1000. https://doi.org/10.1182/blood-2018-09-875013 PMid:30819781
- Martin-Cabrera
P, Jeromin S, Perglerovà K, Haferlach C, Kern W, Haferlach T. Acute
myeloid leukemias with ring sideroblasts show a unique molecular
signature straddling secondary acute myeloid leukemia and de novo acute
myeloid leukemia. Haematologica. 2017;102(4):e125-e128. https://doi.org/10.3324/haematol.2016.156844 PMid:28057736 PMCid:PMC5395122
- Woboda,
D.M., Kanagal-Shamanna, R., Brunner, A.M. et al. Marrow ring
sideroblasts are highly predictive for TP53 mutation in MDS with excess
blasts. Leukemia 36, 1189-1192 (2022). https://doi.org/10.1038/s41375-021-01486-w PMid:34975158
- Kohla
S, Ali E, Amer A, Yousif T, Yassin MA. A Rare Case of Severe Copper
Deficiency in an Infant with Exclusive Breast Feeding Mimicking
Myelodysplastic Syndrome. Case Rep Oncol. 2020 Feb 4;13(1):62-68. https://doi.org/10.1159/000505483 PMid:32110221 PMCid:PMC7036529
- Shehab
Fareed, Firyal Ibrahim, Mohamad Najib Alasafar, et al. Recurrent
sideroblastic anaemia during pregnancy. Authorea. May 09, 2022
[preprint]. https://doi.org/10.22541/au.165209045.56104477/v1
[TOP]