Influence of Sickle Cell Gene on the Allelic Diversity at the msp-1 locus of Plasmodium falciparum in Adult Patients with Severe Malaria
Dilip Kumar Patel1, Ranjeet Singh Mashon1, Prasanta Purohit1, Satyabrata Meher1, Snehadhini Dehury1, Chhatray Marndi1, Kishalaya Das1, Bipin Kishore Kullu1, Siris Patel1 and Padmalaya Das2
1 Sickle Cell Clinic and Molecular
Biology Laboratory, Odisha Sickle Cell Project, Veer Surendra Sai
Medical College, Burla, Sambalpur, Odisha, India.
2 Department of Infectious Diseases, Asian Institute of Public Health, Bhubaneswar, Odisha, India.
Corresponding author: Prasanta Purohit, Senior
Research Fellow, Room No 11, OPD Building. Sickle Cell Clinic and
Molecular Biology Laboratory, V.S.S Medical College and Hospital. PO.
Burla, Dist, Sambalpur, Odisha-768017 (India). Tel: +91-97781-18055;
Fax: +91-663-2432624. E-mail:
prasanta.biochem@gmail.com
Published: August 24, 2015
Received: March 2, 2015
Accepted: July 15, 2015
Mediterr J Hematol Infect Dis 2015, 7(1): e2015050, DOI
10.4084/MJHID.2015.050
This article is available on PDF format at:
This is an Open Access article distributed
under the terms of the Creative Commons Attribution License
(http://creativecommons.org/licenses/by/2.0),
which permits unrestricted use, distribution, and reproduction in any
medium, provided the original work is properly cited.
|
Abstract
Although several studies have
supported that sickle cell trait (HbAS) protects against falciparum
malaria, the exact mechanism by which sickle gene confers protection is
unclear. Further, there is no information on the influence of the
sickle gene on the parasitic diversity of P. falciparum population in
severe symptomatic malaria. This study was undertaken to assess
the effect of the sickle gene on the parasite densities and diversities
in hospitalized adult patients with severe falciparum malaria. The
study was carried out in 166 adults hospitalized subjects with severe
falciparum malaria at Sickle Cell Clinic and Molecular Biology
Laboratory, Veer Surendra Sai Institute of Medical Sciences and
Research, Burla, Odisha, India. They were divided into three groups on
the basis of hemoglobin variants HbAA (n=104), HbAS (n=30) and HbSS
(n=32). The msp-1 loci were genotyped using a PCR-based methodology.
The parasite densities were significantly high in HbAA compared to HbAS
and HbSS. The multiplicity of infection (MOI) and multi-clonality for
msp-1 were significantly low in HbSS and HbAS compared to HbAA. The
prevalence of K1 (p<0 .0001) and MAD20 (p=0.0003) alleles were
significantly high in HbAA. The RO33 allele was detected at a higher
frequency in HbSS and HbAS, compared to K1 and MAD20. Sickle gene was
found to reduce both the parasite densities and diversity of P.
falciparum in adults with severe malaria. |
Introduction
Malaria is the major public health problem in India and accounting
1.1 million of reported cases in the year 2011. The number of malaria
deaths in subjects aged 5 years or older was higher compared to
children younger than 5 years,[1] that calls for a
shifting of malaria control strategies towards adult malaria rather
than focusing only on women and children.[2] There is
little doubt that a highly effective vaccine would play a central role
in preventing these deaths. This requires a better understanding of the
antigenic targets in malaria and the means to overcome the enormous
polymorphism of this targets.[3]
The merozoite
surface protein 1 (MSP-1) is a leading vaccine candidate antigen and
the most abundant surface protein on the blood stage of P. falciparum. msp-1 has extensive genetic diversity.[4]
Moreover, it provides multiple effective evasion and drug resistance
mechanisms for the parasites and presents a major challenge for the
development of an effective malaria vaccine.[5,6] Host polymorphisms like sickle cell gene have been found to influence the population structures of P. falciparum, notably in the genes of P. falciparum those affect the success and virulence of infection.[7]
In malaria endemic regions, the sickle cell gene has attended high
frequency due to its protective effect against severe malaria.[8-9]
Both malaria and sickle cell anemia are major public health problems in
western part of Odisha. The frequency of sickle cell gene in the study
population is 21%.[10] In a hospital-based study in
our institution, we have reported that severe malaria is the second
most common cause of death in HbSS subjects.[11]
Surprisingly there is no information on the influence of sickle cell gene on the parasitic diversity of P. falciparum
population in severe symptomatic malaria in children or adults.
Moreover HbSS, a severe form of chronic hemolytic anemia remains a
source of great suffering to patients, especially in a developing
country like India where the numbers are staggering. Furthermore, when
the patients with HbSS get malaria, there will be a superimposed acute
hemolytic anemia that become a major cause of death in these
patients. Therefore, there is an urgent need to investigate the
association, outcomes and mechanism of interaction between HbSS and
malaria to provide suitable protection against the potentially fatal
threat of P. falciparum
malaria. Given this we undertook this study to find out the influence
of sickle cell gene on the parasitic diversity in the Block 2 region of
the msp-1 in adult subjects with severe malaria.
Materials and Methods
Study Area:
The study was undertaken at the Sickle Cell Clinic and Molecular
Biology Laboratory of Veer Surendra Sai Institute of Medical Sciences
and Research, Burla in the state of Odisha, India. This hospital caters
the population residing in the western part of Odisha state and the
eastern part of Chhattisgarh state. This region has low perennial
transmission of malaria with a high frequency of sickle cell gene (21%)[10] and alpha thalassemia (51%).[12] The state of Odisha contributes to 23% of positive malaria cases, 50% of P. falciparum cases and 15% of malaria-related deaths in India.[13] In the study area located in western part of Odisha, malaria is the foremost public health problem, and P. falciparum accounted for 87.8% of malarial infections.[14]
Study subjects:
Subjects aged 15 years and above, hospitalized in the Department of
Medicine, Veer Surendra Sai Institute of Medical Sciences and Research,
Burla, between July 2007 to September 2008 and diagnosed to have severe
P. falciparum malaria, were included in the study. The severity of P. falciparum malaria was defined as per WHO criteria.[15]
Severe malaria (SM) was categorized into three sub-phenotypes, (1)
Cerebral malaria (CM), (2) Non cerebral severe malaria (NCSM) and (3)
Multi-organ dysfunction (MOD).[16]
Exclusion criteria: Subjects with the following conditions were excluded from the study:
(a) subjects co-infected with other Plasmodium species;
(b) subjects with other sickle cell syndromes like HbSβ-thalassemia, HbSE, HbSC, HbSD-Punjab;
(c) children <15 years of age;
(d) pregnant women;
(e) subjects who refused to consent.
Laboratory Investigations: P. falciparum
examination was made by light microscopy (100 X) of thick blood smears
stained by Giemsa. Parasite densities were counted against 200
leukocytes in thick blood films. All the subjects were screened
for sickle cell gene by sickling slide test. Those found positive were
subjected to agarose gel Hb electrophoresis (pH 8.6) and high
performance liquid chromatography (HPLC) using the VARIANT™ Hemoglobin
Testing System (Bio-Rad Laboratories, Hercules, CA, USA) according to
the manufacturer’s guidelines. A complete blood count was done on an
automated hematology analyzer (Sysmex pocH-100i; Sysmex Corporation,
Kobe, Japan). Biochemical parameters such as serum bilirubin,
creatinine, urea, aspartate transaminase (AST) and alanine transaminase
(ALT) were done in a semi autoanalyzer (Erba Chem 7; Erba Diagnostics
Mannheim GmbH, Mannheim, Germany) as per the manufacturer’s
instructions.
DNA was extracted from 5ml of blood by the standard phenol-chloroform method.[17] Confirmation of P. falciparum infection was done by single step polymerase chain reaction (PCR).[18] Single copy polymorphic gene msp-1 located on chromosome 9 was analyzed by nested PCR for genotyping.[19] Depending on the variable copy number repeats in block 2 of msp-1 gene, three distinct allelic families have been described, namely K1, MAD20 and RO33.
The primary PCR was done with 2µL of DNA as a template, using conserved primers for msp-1. Family specific primers were used for the secondary reactions with 1µl of the primary PCR product. For msp-1,
primer pairs specific for each allelic family (K1, MAD20, and RO33)
were used. The primer sequences and PCR conditions were as described by
Zwetyenga et al.[19] The PCR-amplified gene fragments
of the secondary PCR were electrophoresed on 2% agarose gel and
visualized under Gel documentation system (Model: GelDoc XR; Make:
BioRad Laboratories, USA) after ethidium bromide staining. This allowed
a simultaneous typing of the alleles by size polymorphism and
identification of the allelic family.
MOI and Clonality calculation:
The multiplicity of infection (MOI) was calculated by dividing the
total number of fragments detected in the individual system by the
number of samples positive in the particular system.[20] Multi-clonality was defined as the percentage of subjects showing more than one genetically distinct parasite type for msp-1.
The study was approved by the Institutional Ethical Committee.
Statistical Analysis: Statistical analysis was done using GraphPad InStat Version 3.00 for Windows. The difference in the MOI in the msp-1
family that is (K1, MAD20, and RO33) in the three hemoglobin variants
(HbAA, HbAS, and HbSS) subjects were made using the Kruskal-Wallis
tests. The χ2 test was done to compare the prevalence of msp-1
alleles in the hemoglobin variants and the incidence of various
subphenotypes in severe malaria subjects. P <0.05 was considered
statistically significant.
Results
Of the 198 blood samples collected with suspected severe P. falciparum infection, 166 were finally included in the study (Figure 1). Out of 166 subjects with msp-1
gene polymorphisms, 55.4% were males. The mean age of the subjects was
31.5 ± 10.3 years. There was no statistical difference in the age, sex,
total hemoglobin, total leukocyte count and platelet count in the
subjects among the three different hemoglobin variants. The details of
the three subphenotypes of severe malaria (CM, MOD, and NCSM) are
provided in Table 1. The
parasite densities in the three groups HbAA, HbAS, and HbSS were
8966.6±4368.2, 5531.8±3905.7, and 3699.6±2706.1 respectively. Parasite
densities were significantly high in HbAA compared to HbAS and HbSS (p
<0.0001) and did not change with the age of the subjects (Table 1).
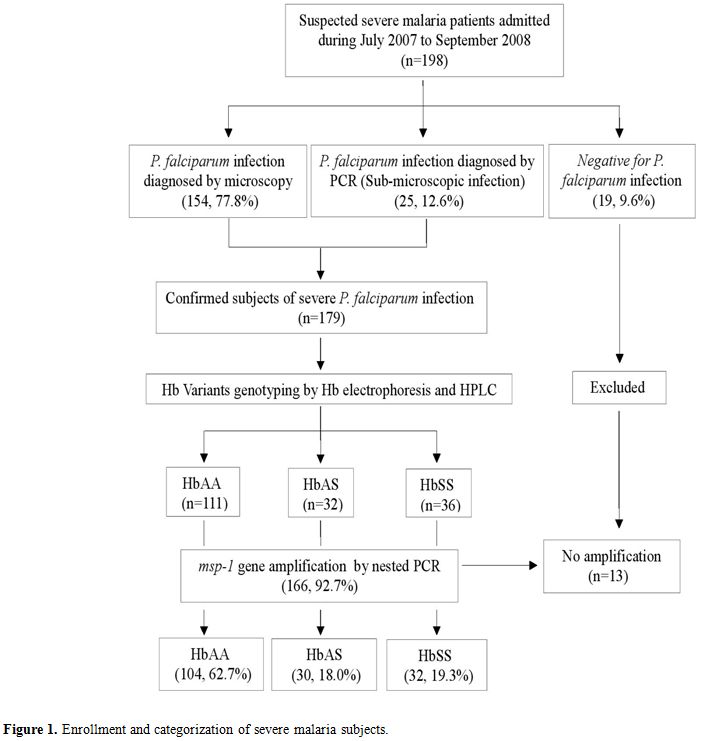 |
Figure
1. Enrollment and categorization of severe malaria subjects. |
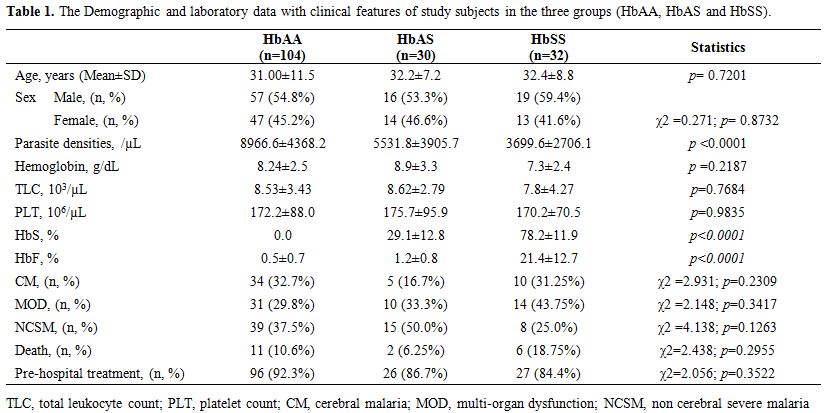 |
Table 1. The Demographic and laboratory data with clinical features of study subjects in the three groups (HbAA, HbAS and HbSS). |
All the three reported families of msp-1 (K1, MAD20, and RO33) were observed among the isolates in the three groups studied. The length variations of msp-1 amplified products in the three groups have been depicted in Table 2.
The multiplicity of infection (MOI) was 3.4, 1.9 and 1.8 in HbAA, HbAS
and HbSS respectively. MOI was significantly low (p=0.03) in HbAS and
HbSS subjects in comparison to HbAA (Table 2).
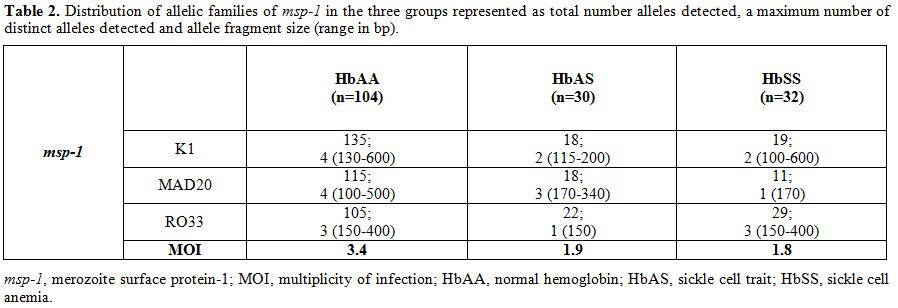 |
Table 2. Distribution of allelic families
of msp-1 in the three groups represented as total number alleles
detected, a maximum number of distinct alleles detected and allele
fragment size (range in bp). |
The prevalence of K1 (χ2=24.28; p<0 .0001) and MAD20 (χ2=16.35;
p=0.0003) alleles were significantly high in HbAA in comparison to HbAS
and HbSS subjects, while the prevalence of RO33 was comparable (χ2=5.11; p=0.0775) in the three groups (Figure 2).
K1, MAD20, and RO33 showed more than 2 PCR products as visualized on
agarose gel as a double band or multiple bands. The PCR amplification
feature of the three allelic families of the msp-1 gene, as visualized by agarose gel electrophoresis, has been depicted in Table 3. The RO33 was polymorphic and presented in up to 3 size polymorphisms, in some of the HbAA and HbSS subjects.
|
Figure
2. Prevalence of three msp-1 alleles in three different genotypes
(HbAA, HbAS and HbSS). msp-1, merozoite surface protein-1; HbAA, normal
hemoglobin; HbAS, sickle cell trait; HbSS, sickle cell anemia. |
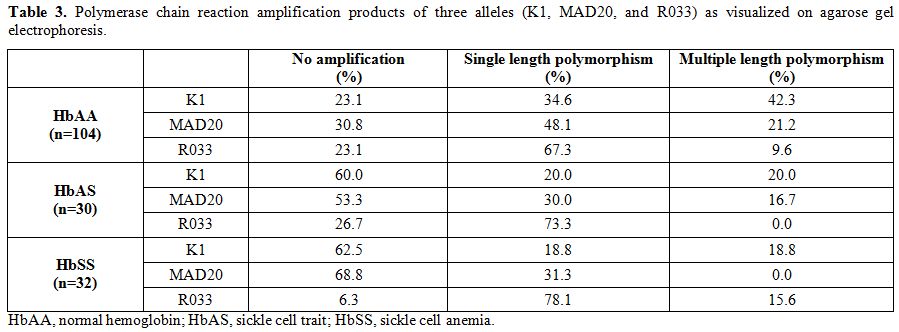 |
Table 3. Polymerase chain reaction
amplification products of three alleles (K1, MAD20, and R033) as
visualized on agarose gel electrophoresis. |
The proportion of multiclonal isolates in the three groups HbAA, HbAS,
and HbSS were 84.6%, 40%, and 37.5% respectively. This multi-clonality
was significantly high in HbAA compared to both HbAS (odds ratio [OR],
0.12; p<0.0001) and HbSS (OR, 0.109; p<0.0001). However, it was similar when compared between HbAS and HbSS (OR, 0.9; p=1.0). The combination of clones detected in the P. falciparum isolate has been illustrated in Table 4.
None of the msp-1
polymorphisms (KI, MAD20, and RO33) were over-represented in any of the
severe malaria sub-phenotypes (CM, MOD and NCSM) in the three groups of
subjects (HbAA, HbAS and HbSS).
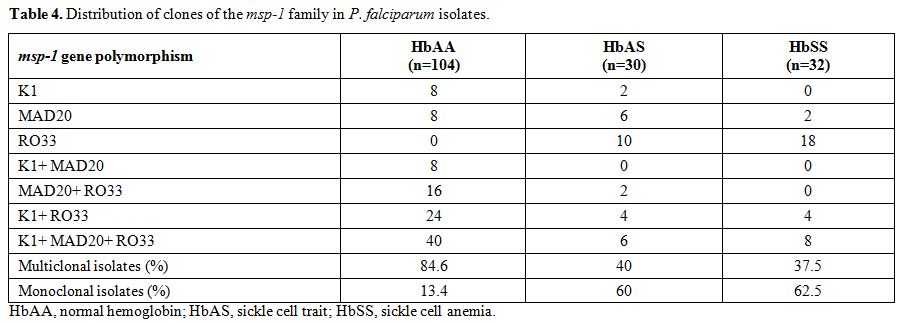 |
Table 4. Distribution of clones of the msp-1 family in P. falciparum isolates. |
Discussion
We
undertook this hospital-based study on 166 subjects in a tertiary care
medical center located in western Odisha. The sizable number of adults
with severe malaria could be due to the low and markedly seasonal
transmission of malaria in this area. In a population with low, erratic
and seasonal parasitic transmission most of the people lack acquired
antiparasitic immunity (premunition), and symptomatic and severe
malaria is found in all age groups including adults.[19]
The various subphenotypes of severe malaria that is CM, MOD and NCSM
were similar in the three groups of subjects (HbAA, HbAS, and HbSS). In
spite of the fact that HbAS confers >90% protection against severe
malaria, we encountered a significant number of HbAS subjects with
severe P. falciparum malaria
(30, 18.07%). This could be due to two reasons that are, the prevalence
of the sickle gene in the western belt of Odisha state is 21%[10]
and the negative epistasis interaction between the malaria protective
effect of alpha thalassemia and HbAS8. In a recent cross-sectional
study, we found that the prevalence of alpha thalassemia in this region
is very high (51%).[12]The
age, sex distribution and baseline laboratory parameters like Hb, TLC
and PLT were similar amongst the three groups. The death rate in HbSS
was higher in comparison to other two groups, although it did not reach
statistical significance. There are several causes of increased
mortality in HbSS subjects infected with P. falciparum malaria, one of which could be increased the parasitic virulence of individual strains reflected by distinct msp alleles.[21,22]The
mean parasite densities of microscopically positive samples in the
present study were significantly lower in HbAS adults when compared to
HbAA. Although several authors have reported low parasite densities in
HbAS during episodes of asymptomatic parasitaemia,[23-25] others have reported no influence.[26,27-30] In symptomatic malaria, the parasite densities have been found to be low in HbAS compared to HbAA.[26,31] Lower parasite densities during symptomatic infection in HbAS could be due to sequestration of P. falciparum
in post-capillary microvessels of the brain and other organs, the
clearing effect of fever on parasitaemia and the increased level of
parasitized HbAS red cells by macrophages.[24,26,32]In
the four different African studies, undertaken in children with
uncomplicated malaria, the parasite densities in HbSS were
significantly lower in comparison to that of HbAA and HbAS.[24,31,33,34]
Ours is the only study where we found significant lower parasite
densities in adult HbSS subjects with severe malaria when compared to
HbAA and HbAS. The various factors that have been found to influence
parasite densities are the age of the subjects,[27] host immune status,[35] transmission intensity,[19,24] disease phenotypes[30] and Hb Variants.[19,24,29,34]
In the present study, the variables like age of the subjects,
transmission intensity and disease phenotypes were similar in the three
groups because all the subjects with severe malaria were of similar age
group, and came from the same geographical area with similar
transmission pattern. So presumably only the sickle cell gene
influenced the parasite densities. Multiple-strain infections are common in P. falciparum
malaria that may overwhelm hosts’ immune systems, leading to resource
competition amongst the parasite clones that might affect the host
morbidity.[36,37] Some studies have found that certain strains of P. falciparum population are associated with more virulent infection.[21,38,39]
Several studies have been undertaken to find out the influence of
sickle cell gene on the multiplicity of infection (MOI) in asymptomatic
malaria in African children with inconsistent results.[23,25,28,29,40-42]
In a lone study conducted in Ghanaian children with symptomatic
uncomplicated malaria, the authors reported that MOI was lower in HbAS
(2.69), and HbSS (2.75) compared to HbAA (3.10). However, this
difference was not statistically significant.[34] The author reported that sickle cell gene had limited influence on the parasite diversity of P. falciparum. MOI has been shown to be reduced in clinical malaria due to the anti-parasitic properties of fever and cytokines.[43]
Alternatively lower MOI could be due to reduced parasite densities or
simply reflect impaired preexisting premonition or indicate abrogation
in symptomatic malaria.[34] The MOI in the present
study was significantly low in HbAS and HbSS genotypes in comparison to
HbAA in adult subjects hospitalized with severe malaria. We studied
another parameter of parasite diversity that is clonality of P. falciparum and found that multiclonal infections were lower in HbAS and HbSS subjects compared to HbAA. Several authors have reported a positive association between parasite diversity with densities of P. falciparum parasite.[23.24,41,44]
The lower MOI and clonality in HbAS and HbSS subjects in our study
could be due to low parasite densities due to the inhibitory effect of
HbS. Besides this other factors including Hb variants that can
influence the parasite diversity are pre-hospital treatment status,
age, transmission intensity, genotyping methods, phenotypes of malaria,
pregnancy and parity, and immune status. In our study, 90% of the
subjects had received pre-hospital treatment with anti-malarial drugs.
The mean age was similar, and all the three groups of subjects came
from areas with similar transmission pattern. We used the same
genotyping method for all the subjects, and none of the subjects was
pregnant. All the subjects in the three groups had severe malaria, and
the subphenotypes of severe malaria were similar. So the only variable
in the three groups which has influenced the parasite diversity is the
presence of sickle cell gene in the HbAS and HbSS state. Studies of msp-1
allelic family distribution in African children with asymptomatic
parasitemia in HbAA and HbAS subjects have reported inconsistent
results.[28,40] So far only one
study in Gabonese children reported no influence of sickle cell gene on
msp alleles family. In the present study, the presence of K1 and MAD20
was significantly higher in HbAA in comparison to HbAS and HbSS
subjects.[34] In HbAA, the prevalence of all the three msp-1
allelic families was similar. However, the RO33 allele was
over-represented in HbAS (73.3%) and HbSS (93.8%). The predominance of
the certain allelic family in Hb variants could be due to reduced
fitness of certain P. falciparum strains in erythrocytes containing HbS.[28]We
studied the association of the various allelic families with phenotypes
of severe malaria in the three groups of subjects. None of the msp-1
allelic families was over-represented in any of the severe malaria
subphenotypes in the three groups of subjects (HbAA, HbAS, and HbSS).
Although some studies have reported over-representation of particular
strain/strains of parasites in subphenotypes of severe malaria, these
results could not be substantiated further in most of the studies.[21,38,39] This study has certain limitations. The use of a single genetic marker (msp-1) may underestimate the genetic diversity of infection.[45] We did not analyze alpha thalassemia in these subjects. Alpha thalassemia influences the susceptibility of P. falciparum population.[8]
A prospective longitudinal field study taking into account the various
host polymorphisms and including sickle cell gene in a significant
number of subjects should be undertaken to overcome these limitations
and derive a conclusive result.In
conclusion parasite densities and all the three parameters of parasite
diversity namely MOI, clonality and allelic family distribution were
significantly reduced in HbAS and HbSS subjects compared to HbAA. Given
this provision of early effective anti-malarial treatment during severe
illness and chemoprophylaxis of HbSS subjects in malaria endemic area
will save valuable lives. Acknowledgment
This study was supported by research funding from Department of
Science and Technology (DST), New Delhi; Indian Council of Medical
Research (ICMR), New Delhi; and Department of Biotechnology (DBT), New
Delhi, Government of India.
References
- World Malaria Report 2013, World Health Organization.
- Murray,
C.J.L., Rosenfeld, L.C., Lim, S.S., et al., Global malaria mortality
between 1980 and 2010: a systematic analysis. Lancet .2012; 379:
413–31. http://dx.doi.org/10.1016/S0140-6736(12)60034-8
- Pierce,
S.K., Miller, L.H. World Malaria Day 2009. What malaria knows about the
immune system that immunologists still do not? The Journal of
Immunolog. 2009;182: 5171-5177. http://dx.doi.org/10.4049/jimmunol.0804153 PMid:19380759 PMCid:PMC2779769
- Holder,
A.A., Patino, J.A.G., Uthaipibull, C., et al., Merozoite surface
protein 1, immune evasion, and vaccines against asexual blood stage
malaria. Parassitologia. 1999; 41: 409-414.PMid:10697894
- Kiwanuka,
G.N. Genetic diversity in Plasmodium falciparum merozoite surface
protein 1 and 2 coding genes and its implications in malaria
epidemiology: a review of published studies from 1997-2007. J Vector
Borne Dis. 2009; 46:1-12. PMid:19326702
- Olasehinde,
G.I., Yah, C.S., Singh, R., et al., Genetic diversity of Plasmodium
falciparum field isolates from south western Nigeria. African Health
Sciences. 2012; 12(3): 355-361. PMid:23382752 PMCid:PMC3557670
- Evans,
A.G., Wellems, T.E. Co-evolutionary genetics of Plasmodium malaria
parasites and their human hosts. Integ and Comp Biol. 2002; 42:401-407.
http://dx.doi.org/10.1093/icb/42.2.401 PMid:21708733
- Williams,
T.N., Mwangi, T.W., Wambua, S., et al., Negative epistasis between the
malaria protective effects of a-thalassemia and the sickle cell trait.
Nat Genet. 2005;37:1253-1257. http://dx.doi.org/10.1038/ng1660 PMid:16227994 PMCid:PMC3521056
- May,
J., Evans, J.A., Timmann, C., et al., Hemoglobin variants and disease
manifestations in severe falciparum malaria. JAMA.
2007;297(20):2020-26. http://dx.doi.org/10.1001/jama.297.20.2220 PMid:17519411
- Purohit,
P., Mashon, R.S., Patel, S., et al., Clinical and molecular
characterization of Hb Hofu in eastern India. Int Jnl Lab Hem.
2014;36:71-76. http://dx.doi.org/10.1111/ijlh.12128 PMid:23889802
- Patel, D.K. Clinical aspects of sickle cell disease in India. 5th Brazilian Symposium for Sickle cell disease and other hemoglobinopathies. Belo Horizonte, Brazil; 2009. http://www.cehmob.org.br/simposio/html/english/download.html
- Purohit,
P., Dehury, S., Patel, S., Patel, D.K., Prevalence of Deletional Alpha
Thalassemia and Sickle Gene in a Tribal Dominated Malaria Endemic Area
of Eastern India. ISRN Hematology. 2014 (2014), ID 745245.
- Epidemiological
Report up to the month of September 2012-2013", Directorate of National
Vector Borne Disease Control Programme (NVBDCP), India. http://nvbdcp.gov.in/Doc/mal-MES-Sept13.pdf.
- Pradhan, M.M. Malaria: Bringing down the burden in Odisha. MMV Stakeholders' Meeting, Delhi (India), 8th November 2012. http://www.mmv.org/sites/default/files/uploads/docs/events/2012/Stakeholder_meeting_presentations/Pradhan_Odisha.pdf
- World Health Organization, 2000. Severe falciparum malaria. Trans R Soc Trop Med Hyg. 94(Suppl 1,S1-90).
- Panda,
A.K., Panda, S.K., Sahu, A.N., et al., Association of ABO blood group
with severe falciparum malaria in adults: case control study and
meta-analysis. Malar J. 2011;10: 309. http://dx.doi.org/10.1186/1475-2875-10-309 PMid:22011404 PMCid:PMC3215225
- Old,
J.M., Varawalla, N.Y., Weatherall, D.J. The rapid detection and
prenatal diagnosis of b thalassaemia in the Asian Indian and Cypriot
populations in the UK. Lancet. 1990;336:834. http://dx.doi.org/10.1016/0140-6736(90)92338-I
- Patsoula,
E., Spanakos, G., Sofianatou, D., et al., A single-step, PCR-based
method for the detection and differentiation of Plasmodium vivax and P.
falciparum. Ann Trop Med Parasitol. 2003;97:15-21. http://dx.doi.org/10.1179/000349803125002535 PMid:12662418
- Zwetyenga,
J., Rogier, C., Tall, A., et al., No influence of age in infection
complexity and allelic distribution in Plasmodium falciparum infections
in Ndiop, a Senegalese village with seasonal, mesoendemic malaria. Am J
Trop Med Hyg. 1998;59:726-735. PMid:9840589
- Joshi,
H., Valecha, N., Verma, A., et al., Genetic structure of Plasmodium
falciparum field isolates in eastern and northeastern India. Malaria J.
2007;6:60. http://dx.doi.org/10.1186/1475-2875-6-60 PMid:17517129 PMCid:PMC1892028
- Ariey,
F., Hommel, D., Scanf, C.L., et al., Association of Severe Malaria with
a Specific Plasmodium falciparum Genotype in French Guiana. The Journal
of Infectious Diseases. 2001;184:237-41. http://dx.doi.org/10.1086/322012 PMid:11424024
- Kun,
J.F., Schmidt-Ott, R.J., Lehman, L.G., et al, Merozoite surface antigen
1 and 2 genotypes and rosetting of Plasmodium falciparum in severe and
mild malaria in Lambarene, Gabon. Trans R Soc Trop Med Hyg. 1998
Jan-Feb;92(1):110-4. http://dx.doi.org/10.1016/S0035-9203(98)90979-8
- Stirnadel,
H.A., Stockle, M., Felger, I., et al., Malaria infection and morbidity
in infants in relation to genetic polymorphisms in Tanzania. Trop Med
Int Health. 1999;4(3):187-193. http://dx.doi.org/10.1046/j.1365-3156.1999.43381.x PMid:10223213
- Branch,
O.H., Takala, S., Kariuki, S., et al., Plasmodium falciparum Genotypes,
Low Complexity of Infection, and Resistance to Subsequent Malaria in
Participants in the Asembo Bay Cohort Project. Infection and Immunity.
2001;69(12):7783-7792. http://dx.doi.org/10.1128/IAI.69.12.7783-7792.2001 PMid:11705960 PMCid:PMC98874
- Kiwanuka,
G.N., Joshi, H., Isharaza, W.K., Eschrich, K. Dynamics of Plasmodium
falciparum alleles in children with normal haemoglobin and with sickle
cell trait in western Uganda. Trans R Soc Trop Med Hyg. 2009;103:87-94.
http://dx.doi.org/10.1016/j.trstmh.2008.07.023 PMid:18789462
- Williams,
T.N., Mwangi, T.W., Wambua, S., et al., Sickle Cell Trait and the Risk
of Plasmodium falciparum Malaria and Other Childhood Diseases. The
Journal of Infectious Diseases. 2005;192:178-86. http://dx.doi.org/10.1086/430744 PMid:15942909 PMCid:PMC3545189
- Achidi,
E.A., Salimonu, L.S., Asuzu, M.C., Berzins, K., Walker, O. Studies on
Plasmodium falciparum parasitemia and development of anemia in Nigerian
infants during their first year of life. Am J Trop Med Hyg. 1996
Aug;55(2):138-43. PMid:8780450
- Ntoumi,
F., Rogier, C., Dieye, A., et al., Imbalanced distribution of
Plasmodium falciparum MSP-1 genotypes related to sickle-cell trait. Mol
Med. 1997;3:581-592. PMid:9323709 PMCid:PMC2230086
- Ntoumi,
F., Mercereau-Puijalon, O., Ossari, S., et al., Plasmodium falciparum:
sickle-cell trait is associated with higher prevalence of multiple
infections in Gabonese children with asymptomatic infections. Exp
Parasitol.1997; 87:39-46. http://dx.doi.org/10.1006/expr.1997.4173 PMid:9287956
- Amodu,
O.K., Olaniyan, S.A., Adeyemo, A.A., et al., Association of the sickle
cell trait and the ABO blood group with clinical severity of malaria in
southwest Nigeria. Acta Tropica. 2012; 123:72- 77. http://dx.doi.org/10.1016/j.actatropica.2012.03.013 PMid:22503377
- Aidoo,
M., Terlouw, D.J., Kolczak, M.S., et al., Protective effects of the
sickle cell gene against malaria morbidity and mortality. Lancet.
2002;359(9314):1311-2. http://dx.doi.org/10.1016/S0140-6736(02)08273-9
- Luzzatto, L. Sickle Cell Anaemia and Malaria. Mediterr J Hematol Infect Dis. 2012;4(1): e2012065. http://dx.doi.org/10.4084/mjhid.2012.065 PMid:23170194 PMCid:PMC3499995
- Komba,
A.N., Makani, J., Sadarangani, M., et al., Malaria as a cause of
morbidity and mortality in children with homozygous sickle cell disease
on the coast of Kenya. Clin Infect Dis. 2009;49(2):216- 222. http://dx.doi.org/10.1086/599834 PMid:19514855 PMCid:PMC2727464
- Mockenhaupt,
F.P., Ehrhardt, S., Otchwemah, R., et al., Limited influence of
haemoglobin variants on Plasmodium falciparum msp1 and msp2 alleles in
symptomatic malaria. Trans R Soc Trop Med Hyg. 2004;98:302-310. http://dx.doi.org/10.1016/j.trstmh.2003.10.001 PMid:15109555
- Mayor,
A., Serra-Casas, E., Rovira-Vallbona, E., et al., Immunoglobulins
against the surface of Plasmodium falciparum-infected erythrocytes
increase one month after delivery. Malar J. 2012;11:130. http://dx.doi.org/10.1186/1475-2875-11-130 PMid:22533971 PMCid:PMC3423004
- de
Roode, J.C., Helinski, M.E., Anwar, M.A., Read, A.F. Virulence and
competitive ability in genetically diverse malaria infections. Proc
Natl Acad Sci U S A. 2005; 102(21):7624-7628. http://dx.doi.org/10.1073/pnas.0500078102 PMid:15894623 PMCid:PMC1140419
- Balmer, O., Tanner, M. Prevalence and implications of multiple-strain infections. Lancet Infect Dis. 2011;11: 868-78. http://dx.doi.org/10.1016/S1473-3099(11)70241-9
- Robert,
F., Ntoumi, F., Angel, G., et al., Extensive genetic diversity of
Plasmodium falciparum isolates collected from patients with severe
malaria in Dakar, Senegal. Trans R Soc Trop Med Hyg. 1996
Nov-Dec;90(6):704-11. http://dx.doi.org/10.1016/S0035-9203(96)90446-0
- Ranjit,
M.R., Das, A., Das, B.P., et al., Distribution of Plasmodium falciparum
genotypes in clinically mild and severe malaria cases in Orissa, India.
Trans R Soc Trop Med Hyg. 2005; 99(5): 389-95. http://dx.doi.org/10.1016/j.trstmh.2004.09.010 PMid:15780346
- Konate,
L., Zwetyenga, J., Rogier, C., et al., Variation of Plasmodium
falciparum msp1 block 2 and msp2 allele prevalence and of infection
complexity in two neighbouring Senegalese villages with different
transmission conditions. Trans R Soc Trop Med Hyg. 1999; 93(Suppl
1):21-28. http://dx.doi.org/10.1016/S0035-9203(99)90323-1
- Vafa,
M., Troye-Blomberg, M., Ancgang, J., et al., Multiplicity of Plasmodium
falciparum in asymptomatic children in Senegal: relation to
transmission, age and erythrocyte variants. Malaria J. 2008;7:17. http://dx.doi.org/10.1186/1475-2875-7-17 PMid:18215251 PMCid:PMC2267475
- Koussounda,
F.K., Malonga, V., Mayengue, P.I., et al., Genetic polymorphism of
merozoit surface protein 2 and prevalence of K76T pfcrt mutation in
Plasmodium falciparum field isolates from Congolese children with
asymptomatic infections. Malaria J. 2012;11:105. http://dx.doi.org/10.1186/1475-2875-11-105 PMid:22463364 PMCid:PMC3349535
- Kwiatkowski, D. Cytokines and anti-disease immunity to malaria. Res Immunol. 1991;142(8): 707-712. http://dx.doi.org/10.1016/0923-2494(91)90154-B
- Felger,
I., Irion, A., Steiger, S., Beck, H.P. Genotypes of merozoite surface
protein 2 of Plasmodium falciparum in Tanzania. Trans R Soc Trop Med
Hyg. 1999;93 Suppl 1:3-9. http://dx.doi.org/10.1016/S0035-9203(99)90320-6
- Kiwuwa,
M.S., Ribacke, U., Moll, K., et al., Genetic diversity of Plasmodium
falciparum infections in mild and severe malaria of children from
Kampala, Uganda. Parasitol Res. 2013;112:1691-1700. http://dx.doi.org/10.1007/s00436-013-3325-3 PMid:23408340 PMCid:PMC3597336