Lucrezia Laterza1,
Gianenrico Rizzatti1, Eleonora Gaetani1, Patrizia
Chiusolo2 and Antonio Gasbarrini1
1 Fondazione
Policlinico A. Gemelli,
UOC of Internal Medicine, Gastroenterology and Liver Diseases.
L.go Gemelli, 8 Rome, Italy
2 Fondazione Policlinico A. Gemelli, Institute of
Haematology, L.go Gemelli, 8 Rome, Italy
Published: May 1, 2016
Received: February 22, 2016
Accepted: April 10, 2016
Mediterr J Hematol Infect Dis 2016, 8(1): e2016025, DOI
10.4084/MJHID.2016.025
This article is available on PDF format at:
This is an Open Access article distributed
under the terms of the Creative Commons Attribution License
(https://creativecommons.org/licenses/by-nc/4.0),
which permits unrestricted use, distribution, and reproduction in any
medium, provided the original work is properly cited.
|
Abstract
Gut microbiota has gained
increasing
interest in the pathogenesis of immune-related diseases. In this
context, graft-versus-host disease is a condition characterized by an
immune response which frequently complicates and limits the outcomes of
hematopoietic stem cell transplantations. Past studies, carried mostly
in animals, already supported a relationship between gut microbiota and
graft-versus-host disease. However, the possible mechanisms underlying
this connection remain elusory. Moreover, strategies to prevent
graft-versus-host disease are of great interest as well as the
potential role of gut microbiota modulation. We reviewed the role
of gut microbiota in the development of immune system and its
involvement in the graft-versus-host disease, focusing on data
available on humans.
|
Introduction
Microbiota is the complex system of bacteria, archaea, viruses and
fungi living in several body niches, such as skin, vagina, nose and
mouth. However, the majority of microorganisms live in the digestive
tract. Gut microbiota should be considered a real organ, accounting 100
times more genes than the host and being responsible for multiple
functions and in particular of the metabolic and immune homeostasis.[1]
Recent
studies demonstrated that gut microbiota is only the first layer of a
multilayer barrier separating our organism from the content of
intestinal lumen and, thus, from the external environment: the
so-called “gut barrier”. This barrier is composed, beyond microbiota,
by the mucus layer on the epithelial cells, the epithelial cells
themselves, the immune cells harboring in the submucosa and by the
bidirectional interactions between all these layers (Figure 1).
Its integrity is essential to maintain the homeostasis, and its
disruption has been associated with many gastrointestinal and
extragastrointestinal diseases. Whereas the role of gut barrier
disruption appears clear in gastrointestinal disorders, its role in
extragastrointestinal diseases could be harder to understand. The basis
of this role should be searched in the complex function of immune
stimulation/tolerance that gut microbiota exerts.
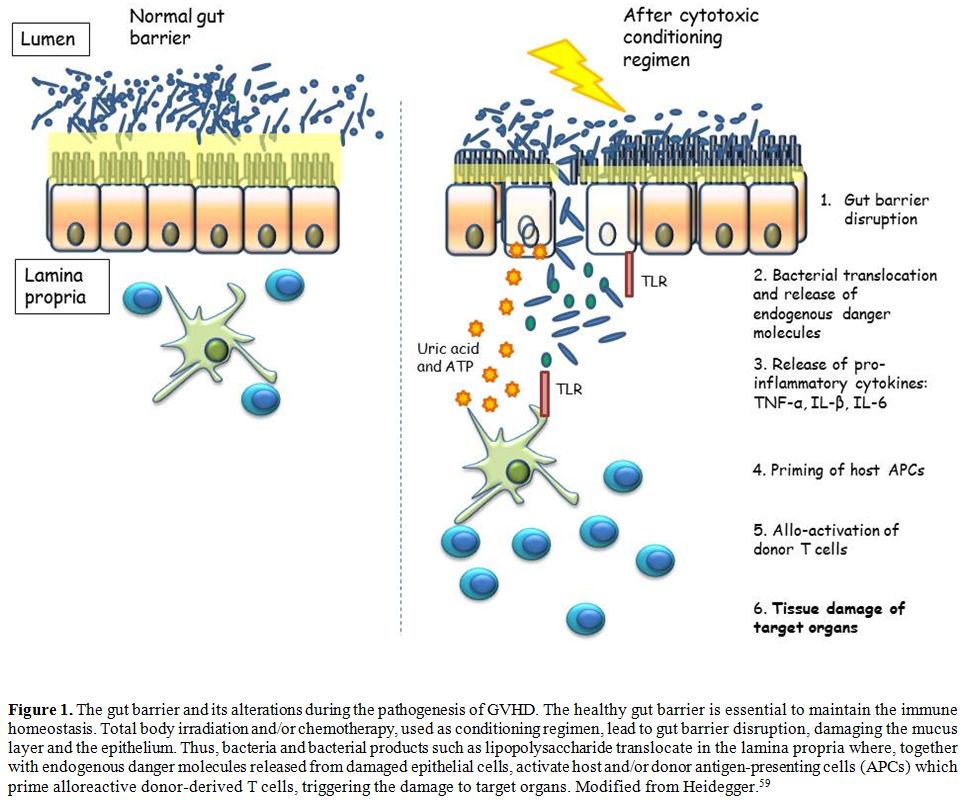 |
Figure
1. The gut barrier and its alterations during the pathogenesis of GVHD.
The healthy gut barrier is essential to maintain the immune
homeostasis. Total body irradiation and/or chemotherapy, used as
conditioning regimen, lead to gut barrier disruption, damaging the
mucus layer and the epithelium. Thus, bacteria and bacterial products
such as lipopolysaccharide translocate in the lamina propria where,
together with endogenous danger molecules released from damaged
epithelial cells, activate host and/or donor antigen-presenting cells
(APCs) which prime alloreactive donor-derived T cells, triggering the
damage to target organs. Modified from Heidegger.[59] |
Hematopoietic stem cell transplantation (HSCT) is a
potentially curative therapy for many diseases, mostly hematological,
otherwise associated with a poor prognosis. Unfortunately, the
widespread use of this treatment is often restricted by the development
of graft-versus-host disease (GVHD) a condition in which
immunocompetent donor T cells attack host tissues in immunocompromised
patients, constituting one of the leading causes of non-relapse
mortality.[2] GVHD depends on several factors, such as
age, conditioning regimen, hematopoietic graft source and prophylaxis.
The traditional classification of GVHD is based on the timing of onset:
acute (aGVHD), within the first 100 days after HSCT, and chronic
(cGVHD), after the first 100 days. However, beyond the temporal
criterion, aGVHD and cGVHD are different diseases, with characteristic
clinical presentation, diagnostic criteria, and tissue pathology.
Systemic inflammation and tissue disruption predominate in aGVHD,
whereas the immune dysregulation leading to several infections is the
prevalent presentation in cGVHD.[3] Thus, the
characteristic clinical manifestations became the diagnostic features
instead of the time of the onset, based on National Institutes of
Health (NIH) consensus criteria.[4]
In particular, in this review we discuss the role of gut microbiota in
the GVHD, focusing on data on humans.
The Healthy Gut Microbiota
In the last years, the increasing interest on human gut microbiota
led to large-scale attempts to characterize it. The association of
traditional cultural techniques with new molecular techniques based on
the analysis of the small subunit ribosomal RNA (SSU rRNA) gene
sequences as phylogenetic markers made bacteria the most known
components of gut microbiota, identifying more than 1000 species.
Bacteria together with Archaea and Eukaryota constitute the three
kingdoms in which life is classified. Bacteria are subclassified in
many phyla (plural of phylum, major taxonomic division that contains
one or more classes, Box 1),
but only a few phyla are mostly represented, accounting for more than
160 species, and, among them, Firmicutes (consisting mainly of
Gram-positive clostridia) and Bacteroidetes (consisting mainly of
Gram-negative bacteria) are prevalent.[1,5]
These two phyla, together with the less represented Actinobacteria and
Proteobacteria are not only the most abundant, but also include
the most diverse microorganisms in
the adult gastrointestinal tract. Other represented phyla
are Verrucomicrobia, Lentisphaerae, Synergistetes, Planctomycetes,
Tenericutes and the Deinococcus-Thermus group, Melainabacteria, and
Gemmatimonacete. Regarding the other two kingdoms, the Euryarchaeota,
including the highly represented methanogens, are the most represented
Archaea, whereas, among the Eukarya, some Candida spp are the most
prevalent.
The earliest years of life are essential for the
development of individual microbiota that depends on several factors,
such as maternal and family members microbiota, kind of delivery,
breastfeeding and early exposure to antibiotics. After this phase,
individual microbiota composition is stable in the adult life for
decades, and it may be the same also for the entire lifetime unless
perturbing factors occur, such as antibiotic therapies or infections.[6]
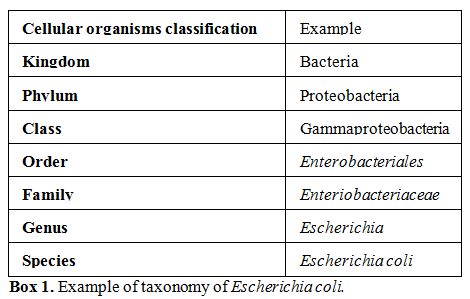 |
Box 1. Example of taxonomy of Escherichia coli. |
The Role of Gut Microbiota in the Immune Regulation
The correct development of gut microbiota is strictly related to the
healthy maturation of the immune system, and both develop in the first
2 years of life. In fact gut microbiota constitutes a stimulus that
drives the development of the immune system in its capacity to react to
pathogens and in the induction and maintenance of the tolerance
process. On the other side, immune dysregulation can induce an
alteration in gut microbiota.[7,8] The importance of
this bidirectional relationship has been highlighted by data from
germ-free (GF) animals that showed reduced development of both innate
and adaptive immunity with increased susceptibility to microbial
infections.[9,10]
The integrity of the gut barrier is the basis of the healthy
stimulation of the immune system by microbiota.
In
fact, the continuous stimulation by luminal commensal antigens should
be regulated to avoid the over-stimulation of the immune system. This
is warranted by the presence of a physical barrier between gut
microbiota and host immune cells, composed of epithelial cells and the
mucus layer above them. In particular, the mucus layer consists of an
inner and an outer layer, but whereas the outer one is colonized by
large numbers of bacteria, the inner one, thicker than the outer one,
constitutes a barrier for them.[11,12] Furthermore,
even innate lymphoid cells[13,14] and IgAs[15]
contribute to reduce the penetration of microorganisms through the
epithelial cells and their presentation to the immune system.
Microbiota is essential for the correct development of both innate and
adaptive immune response.
Conversely, microbiota needs a healthy
immune system to correct its development. In fact, for example, the
deficit in IgA response alters the composition of microbiota.[16-18]
Microbiota and the Innate Immune Response
Gut
microbiota could regulate lamina propria phagocytes and, in particular,
it could increase the production of pro-IL1β in resident macrophages[19] and neutrophils,[20]
that could be rapidly activated in IL1β in response to pathogens.
Microbiota could also influence systemic neutrophils response enhancing
their bactericidal activity triggering the NOD1 signaling through
peptidoglycan stimulation.
Microbiota and the Adaptive Immune Response
Data
from germ-free (GF) animals demonstrated that when the microbiota is
absent, there is a shift through a T-helper (Th)2 response, due to a
reduced number of Th1 and Th17 cells, which could be reversible in case
of colonization of the gut by flora. In particular, in the small
intestine Th17 cells could be stimulated mainly by segmented filamentous bacteria
(SFB), species belonging to commensal Clostridia-related bacteria,[21-24] and Lactobacillus
johnsonii.[25]
Beyond
T cells, also B cells and immunoglobulins production are influenced by
microbiota. In fact, the intestinal mucosa is essential to the correct
development of B cells as well as fetal liver and bone marrow, and
microbiota is able to regulate intestine-specific B-cell receptor.[26,27]
In fact, the presence of commensal microorganisms in the gut stimulates
gut-associated lymphoid tissues (GALTs), such as both Peyer’s patches
and isolated lymphoid follicles.[28-30] The
continuous stimulation induces germinal center formation in isolated
lymphoid follicles and Peyer’s patches and IgA production, differently
from systemic lymphoid organs where germinal center formation does not
occur under physiological condition, but only after a specific- i.e.
infectious- stimulation.[31] In fact, microbial
products are required to stimulate the germinal centers in lymphoid
follicles and IgA production, in particular through Nucleotide-binding
oligomerization domain-containing protein (NOD)1-mediated signaling.[18,32,33]
Tolerance Education by Microbiota
Colonic FoxP3+ T regulatory (Treg)
cells are strongly influenced by the presence of gut microbiota. In
fact, they are reduced in colonic lamina propria in the absence of gut
microbiota stimulation, whereas the presence of gut microbiota is less
relevant for Treg of the small intestine or mesenteric lymph nodes.[34,35] In particular, murine data demonstrated that Clostridia and Bacteroides fragilis could be the
most powerful inducers of Treg,[34-39] probably
working through different mechanisms which could be dependent and
independent from toll-like receptors (TLRs) signaling. Among
TLRs-independent pathways, short-chain fatty acids (SCFAs) - bacterial
metabolites deriving from carbohydrates fermentation, including
acetate, propionate, isobutyrate and butyrate- seem to be able to
increase the acetylation of the Foxp3 locus, increasing the number of
Treg directly or, indirectly, increasing the production of TGFβ in the
intestinal epithelium.[36,40-42]
Furthermore, SCFAs induced the expression of the receptor GPR15,
responsible for recruitment of Treg in the large intestine.[40-50] Similarly, the folic acid produced by colonic
microorganisms could increase the survival of Treg cells.[51]
Furthermore, gut microbiota could stimulate the production of the
anti-inflammatory cytokine IL10 by intestinal macrophages.[52]
The Allogenic Transplant and the Graft-versus-Host
Disease
Every year, more than 39000[53]
HSCT are performed only in Europe for an ever expanding number of
neoplastic and non-neoplastic diseases, in particular for hematological
conditions such as leukemias and lymphomas.[54]
Nevertheless,
HSCT is still limited by the development of GVHD, a condition that
results from the interaction between the host cells which are targeted
by the transplanted donor immune cells, primarily T cells.[55]GVHD
was historically classified in acute and chronic, respectively, if the
onset of symptoms was before or after 100 days. However recent
advantages questioned these definitions, and current consensus states
that clinical features define GVHD as acute or chronic.[4]
aGVHD[56]
occurs mainly in the skin, GI, and liver. GI manifestations of aGVHD
include secretory diarrhea, vomiting, abdominal pain and, in severe
cases, bleeding. The severity of aGVHD is classified in four grades on
the basis of the involvement of the organs mentioned above.[57]
On the other hand, cGVHD manifestations are typically variable, and
many organs can be involved, frequently with autoimmune-like diseases
characteristics.[58]
GVHD Pathogenesis and the Role of Gut Microbiota
The
mechanisms leading to GVHD are usually divided into steps: organ
damage, priming of the immune response, activation of T cells and
destruction of target organs by mean of the activated immune cells[2,57,59] (Figure 1).
The incidence of GVHD is positively correlated with the degree of human
leucocyte antigen (HLA) mismatch as the histocompatibility antigens are
the main proteins recognized by donor immune cells.[60]
The connection between GVHD and microbiota was firstly suggested in
pioneering studies in mice.[61,62]
However, studies in humans are still scant and characterized by small
sample sizes. These studies mainly investigated variations in the
gastrointestinal microbiota before and after HSCT and the impact of its
composition on the transplant outcomes (Table
1).
|
Table 1. Summary of human studies assessing
gastrointestinal microbiota in Graft versus Host Disease. |
Taur
et al. demonstrated that there is a marked reduction after HSCT in the
microbiota diversity which leads to the selection of a limited number
or, even, of a single “dominating” bacterial genus. Interestingly,
patients who developed intestinal domination showed an increased risk
of bacteremia which was frequently caused by the same identified
“dominating” bacteria.[63] The authors also described
the effects of different antibiotics on the development of specific
bacterial prevalences: for example, fluoroquinolones reduced the risk
of gram-negative bacteremia by decreasing proteobacterial domination.[63]
Given
these data and considering the already mentioned dramatic impact of
GVHD on survival, it is not surprising that microbiota diversity was
also found to be an independent risk factor for mortality in patients
undergoing HSCT.[66]
Consequent
studies focused on the analysis of bacterial composition, researching
if specific genera or species could be more implicated than others in
the development of GVHD. For example, analysis of bacterial genera
found that the abundance of a specific genus, namely Blautia (which belong to the Clostridia class), is associated
with GVHD-related mortality.[67]
Although it was not possible to demonstrate causality in this study,
these data may represent a starting point for the development of a GVHD
mortality biomarker in the near future.
Other
studies investigated variations of the microbiota in relation to the
development of GVHD, the second most common cause of mortality in the
context of allogeneic HSCT.[64] In particular, the
onset of GVHD seems to be associated with a progressive reduction of
the microbiota diversity with a relative increase in Lactobacillales
and a relative decrease in Clostridiales.[65]
Noteworthy, these findings are consistent with those in mice,
suggesting that animal studies may, at least, guide the research in
humans.
Similarly,
other authors reported that there is an increase after HSCT in the
relative abundance of enterococci that was persistent and more
pronounced in adult patients with active GVHD.[68]
Similar results have been obtained in children by Biagi et al., who
analyzed fecal samples collected from 10 children before HSCT and three
times in the following 100 days. After HSCT, a profound alteration of
the gut ecosystem occurred in all children, with the loss of about 30%
in average of alpha diversity -a measure of diversity within a
population in terms of number and distribution- compared to pre-HSCT
samples. However, the last samples collected showed a minor degree of
difference compared to pre-HSCT specimens, suggesting a natural trend
to recover after the disturbance caused by the HSCT. The fecal amount
of short-chain fatty acids (SCFA) followed the variations of
microbiota: it decreased by 76% after HSCT, being propionate the most
reduced (mean loss 86%), and trend to recover distancing the HSCT.
Although these differences are common in patients with and without
aGVHD, the 5 children who developed aGVHD also showed an overgrowth of Enterococcus and Clostridiales and a corresponding
decrease of Faecalibacterium
and Ruminococcus. At phylum
level, patients with aGVHD showed a drop in Firmicutes
abundance after HSCT but, distancing the HSCT, they showed higher
abundance than the initial one, whereas they demonstrated a lower
abundance of Bacteroidetes
compared to non-aGVHD patients. Even if alterations of gut microbiota
induced by conditioning regimen and HSCT seem to be crucial to the
pathogenesis of GVHD, the pre-HSCT characteristics of gut microbiota
could also play a major role. In fact, children who developed aGVHD
showed lower diversity and richness before HSCT compared to the other
patients and, in particular, they demonstrated a lower abundance of Bacteroides and Parabacteroides, whose abundance
positively correlated with the concentration of propionate and SCFA.[56]
Our
group identified that the conditioning regimen, starting from the same
baseline microbiota composition, promotes changes in the microbiome,
which are different between Autologous (auto-) and Allogeneic Stem Cell
Transplantation (allo-SCT). After auto-SCT we documented an increase of
Proteobacteria (Klebsiella, Proteus, Acinetobacter,
Haemophilus, Pseudomonas, Enterobacteriaceae) and a reduction of
Bacteroidetes (Bacteroides, Saprospirae, Prevotella).
After allo-SCT, instead, there was an increase of Bacteroidetes and a reduction of Firmicutes (Bacilli, Lactobacilli, Clostridium,
Enterococci, Streptococci). Moreover, patients who developed
GVHD harbored more Firmicutes
and Proteobacteria and fewer
Bacteroidetes than patients
without this complication. In patients with gut GVHD, Proteobacteria were more
represented than in patients with liver or skin involvement.[69]
Collectively,
these studies showed that the intestinal microbiota is heavily affected
by HSCT, being the principal finding, reported in all studies, the
reduction in the overall bacterial diversity. At the same time, some
studies reported specific alterations which are interestingly
correlated with the development of the major complications of HSCT,
such as bacteremia and GVHD. While a causative role of the microbiota
in these conditions is yet to be demonstrated, these and future studies
may give a better comprehension of the complex mechanisms underlying
HSCT and GVHD, ultimately allowing better outcomes.
New Perspectives: the Role of Paneth Cells and
Genetic Modifiers of Gut Microbiota
Recently,
researchers focused on Paneth cells in an attempt to find a mechanistic
relation between microbiota and GVHD. Paneth cells secrete
antimicrobial peptides such as alpha-defensins which contribute to the
regulation of the GI microbiota. During GVHD, Paneth cells appear to be
damaged with a consequent reduction in alpha-defensins production.[70]
Noteworthy, alpha-defensins activity is directed mostly toward
non-commensal bacteria, thus decreased levels of these peptides lead to
a reduction of commensal bacteria and, intuitively, to an impairment in
their beneficial effects.
A
subsequent study investigated if Paneth cells number may correlate with
the severity, response to treatment and survival of GVHD. Authors found
that Paneth cells number was inversely correlated with the clinical
severity stage with a strong correlation between the two parameters.
Response to treatment at 4 weeks was also found to be positively
correlated with Paneth cells number, being highest in patients with a
complete response and lowest in patients who did not respond. Finally,
a threshold of 4 Paneth cells per high power field (HPF) was found to
discriminate between high and low-risk patients regarding non-relapse
mortality (NRM), with also a significant difference in the overall
survival.[71]
Similarly,
Ferrara et al. demonstrated that a specific lectin secreted by Paneth
cells, namely regenerating islet-derived 3-alpha (REG3alpha), has
diagnostic value in acute GI GVHD permitting to differentiate between
GVHD-related diarrhea and other causes of diarrhea.[72]
The authors also demonstrated a prognostic value of REG3alpha in GVHD,
in particular, a positive correlation between plasma levels and NRM was
found. This result may appear in contrast with the previous data, in
particular with the evidence supporting a protective role of Paneth
cells. However, the authors hypothesized that the GI mucosal barrier
disruption which occurs in GVHD permits to the nearby Paneth cells
secretions to enter the bloodstream.[72]
Similarly,
there is an increasing interest in the role of the Fucosyltransferase 2
(FUT2) gene, a genetic modifier of the GI microbiota which seems to be
associated with different GI diseases.
Various
antigens are expressed in the intestinal mucin layer, for example, ABH
antigens are oligosaccharides that constitute a site of attachment and
a carbon source for intestinal bacteria.[73] Their
expression is regulated by an enzyme which in humans is encoded by the
FUT2 gene.[74]
Polymorphisms in the FUT2 gene are correlated with alteration of the GI
microbiota both in the compositional and functional level.[75]
Recently, homozygosity for the loss-of-function alleles (non-secretors,
A/A genotype) was demonstrated to be associated with increased
susceptibility to Crohn’s disease.[76] Rayes et
al. also showed that FUT2 polymorphisms influence the risk of GVHD and
bacteremia in the context of HSCT. Specifically, the Authors found that
there was a reduced risk of acute GVHD with A/A genotype
(non-secretors) and an increased risk with the G/G genotype (secretors)
while an increased risk for bacteremia was found with A/A and A/G
(secretors) genotypes.[73]
Gut Microbiota Modulation as a Preventive Strategy
Against GVHD
Considering
the major role of gut microbiota in the pathogenesis of GVHD, its
modulation with prebiotic, probiotic and antibiotic could be a strategy
to reduce the incidence of GVHD.
Some
studies reported reduced numbers and less severe GVHD after the use of
broad-spectrum antibiotics to “decontaminate” the gastrointestinal
tract.[
77,78] However, GI decontamination fell into
disuse in the 1990s mostly because of heterogeneous successful
decontamination rates, high costs and lack of corroborating data of its
utility.[
79] The reasons behind the failure of total
gut decontamination in the clinical setting are still unknown. However,
many Authors hypothesized that an explanation may be that this practice
affects the microbiota as a whole, without distinction between “good”
bacteria and pathogens.[
66,67,
80]
In fact, as discussed above, there is evidence that bacteria diversity
is the cornerstone of the “healthy” microbiota while a decreased
diversity is often found in many GI and extragastrointestinal diseases.[
81]
Probiotics
drew growing interest in the world of gut microbiota modulation and
suggested in murine models that they could be effective in decreasing
the aGVHD severity.[
65,
82] However,
the use of probiotics in immunosuppressed patients is limited by
possible safety issues. Ladas et al. evaluated the safety of the
administration of
Lactobacillus
plantarum
during the conditioning regimen and the post-HSCT neutropenic period in
30 children and adolescents. The majority of patients (70%) did not
develop GVHD. Three patients died within 100 days from the HSCT, but
the causes of death are judged unrelated to probiotics. Furthermore, no
episodes of
Lactobacillus plantarum
bacteremia were observed. Even if these results are preliminary, they
laid the foundation for larger clinical trials to evaluate the efficacy
and the safety of probiotics in prevention and treatment of GVHD.
Prebiotics
are defined as nondigestible food components that are able to modulate
the intestinal microbiota with a possible beneficial effect on the
human body.[
83] In particular, oligosaccharides
represent an important fraction of human milk and are known to exert a
prebiotic effect.[
84]
Modulation of the microbiota is also achieved indirectly, as
oligosaccharides can prevent adhesion of enteropathogens acting as
soluble decoys.[
85] Both these mechanisms indirectly
reduce inflammation,[
86] even if recent evidence
suggest that oligosaccharides also have a direct inhibitory effect on
inflammation.[
87-89]
Fecal
microbiota transplantation (FMT), the infusion of feces from a healthy
donor into the gut of a recipient patient, was recently proven to be
safe and effective in
Clostridium
difficile infection after HSCT when conventional therapy failed.[
90,91]
In fact, patients undergoing HSCT are exposed, due to antibiotic
prophylaxis and to the procedure itself, to colonization by multi-drug
resistant bacteria with a negative impact on the main transplant
outcomes, such as overall survival and non-relapse mortality, but also
on the development of clinically relevant aGVHD.[
92]
Interestingly
the same authors found, in a preliminary study, that FMT was able to
eradicate resistant bacteria harbored in the gut of an
immunocompromised patient affected by multiple myeloma.[
93]
While
further studies are awaited, these data suggested that FMT may
represent, in the near future, a novel strategy to modulate the gut
microbiota with a possible impact on GVHD.
Conclusions
Gut
microbiota and its continuous stimulation of immune system are
essential for the development and the “maintenance” of a healthy
gut. HSCT-related procedures could alter this balance laying the
foundation for the development of GVHD. The possibility of modulation
of gut microbiota as a preventive or curative strategy for GVHD is
intriguing and should be developed in the future, to reduce the
morbidity and mortality of this condition.
References
- Marchesi JR, Adams DH, Fava F, Hermes GD,
Hirschfield GM, Hold G, Quraishi MN, Kinross J, Smidt H, Tuohy KM,
Thomas LV, Zoetendal EG, Hart A. The gut microbiota and host health: a
new clinical frontier. Gut 2016; 65: 330-339. http://dx.doi.org/10.1136/gutjnl-2015-309990
- Pasquini
MC. Impact of graft-versus-host disease on survival. Best practice
& research Clinical haematology 2008; 21: 193-204. http://dx.doi.org/10.1016/j.beha.2008.02.011
- Blazar
BR, Murphy WJ, Abedi M. Advances in graft-versus-host disease biology
and therapy. Nature reviews Immunology 2012; 12: 443-458. http://dx.doi.org/10.1038/nri3212
- Filipovich
AH, Weisdorf D, Pavletic S, Socie G, Wingard JR, Lee SJ, Martin P,
Chien J, Przepiorka D, Couriel D, Cowen EW, Dinndorf P, Farrell A,
Hartzman R, Henslee-Downey J, Jacobsohn D, McDonald G, Mittleman B,
Rizzo JD, Robinson M, Schubert M, Schultz K, Shulman H, Turner M,
Vogelsang G, Flowers ME. National Institutes of Health consensus
development project on criteria for clinical trials in chronic
graft-versus-host disease: I. Diagnosis and staging working group
report. Biology of blood and marrow transplantation: journal of the
American Society for Blood and Marrow Transplantation 2005; 11:
945-956. http://dx.doi.org/10.1016/j.bbmt.2005.09.004
- Rajilic-Stojanovic
M, de Vos WM. The first 1000 cultured species of the human
gastrointestinal microbiota. FEMS microbiology reviews 2014; 38:
996-1047. http://dx.doi.org/10.1111/1574-6976.12075
- Shreiner
AB, Kao JY, Young VB. The gut microbiome in health and in disease.
Current opinion in gastroenterology 2015; 31: 69-75. http://dx.doi.org/10.1097/MOG.0000000000000139
- Lozupone
CA, Rhodes ME, Neff CP, Fontenot AP, Campbell TB, Palmer BE.
HIV-induced alteration in gut microbiota: driving factors,
consequences, and effects of antiretroviral therapy. Gut microbes 2014;
5: 562-570. http://dx.doi.org/10.4161/gmic.32132
- Salas JT, Chang TL. Microbiome in human
immunodeficiency virus infection. Clinics in laboratory medicine 2014;
34: 733-745. http://dx.doi.org/10.1016/j.cll.2014.08.005
- Jain
N, Walker WA. Diet and host-microbial crosstalk in postnatal intestinal
immune homeostasis. Nature reviews Gastroenterology & hepatology
2015; 12: 14-25. http://dx.doi.org/10.1038/nrgastro.2014.153
- Tomkovich S, Jobin C. Microbiota and host immune
responses: a love-hate relationship. Immunology 2016; 147: 1-10.
http://dx.doi.org/10.1111/imm.12538
- Johansson
ME, Phillipson M, Petersson J, Velcich A, Holm L, Hansson GC. The inner
of the two Muc2 mucin-dependent mucus layers in colon is devoid of
bacteria. Proceedings of the National Academy of Sciences of the United
States of America 2008; 105: 15064-15069. http://dx.doi.org/10.1073/pnas.0803124105
- Bergstrom
KS, Kissoon-Singh V, Gibson DL, Ma C, Montero M, Sham HP, Ryz N, Huang
T, Velcich A, Finlay BB, Chadee K, Vallance BA. Muc2 protects against
lethal infectious colitis by disassociating pathogenic and commensal
bacteria from the colonic mucosa. PLoS pathogens 2010; 6: e1000902. http://dx.doi.org/10.1371/journal.ppat.1000902
- Vaishnava
S, Behrendt CL, Ismail AS, Eckmann L, Hooper LV. Paneth cells directly
sense gut commensals and maintain homeostasis at the intestinal
host-microbial interface. Proceedings of the National Academy of
Sciences of the United States of America 2008; 105: 20858-20863. http://dx.doi.org/10.1073/pnas.0808723105
- Sonnenberg
GF, Monticelli LA, Alenghat T, Fung TC, Hutnick NA, Kunisawa J, Shibata
N, Grunberg S, Sinha R, Zahm AM, Tardif MR, Sathaliyawala T, Kubota M,
Farber DL, Collman RG, Shaked A, Fouser LA, Weiner DB, Tessier PA,
Friedman JR, Kiyono H, Bushman FD, Chang KM, Artis D. Innate lymphoid
cells promote anatomical containment of lymphoid-resident commensal
bacteria. Science 2012; 336: 1321-1325. http://dx.doi.org/10.1126/science.1222551
- Strugnell
RA, Wijburg OL. The role of secretory antibodies in infection immunity.
Nature reviews Microbiology 2010; 8: 656-667.10.1038/nrmicro2384 http://dx.doi.org/10.1038/nrmicro2384
- Fagarasan
S, Muramatsu M, Suzuki K, Nagaoka H, Hiai H, Honjo T. Critical roles of
activation-induced cytidine deaminase in the homeostasis of gut flora.
Science 2002; 298: 1424-1427. http://dx.doi.org/10.1126/science.1077336
- Wei
M, Shinkura R, Doi Y, Maruya M, Fagarasan S, Honjo T. Mice carrying a
knock-in mutation of Aicda resulting in a defect in somatic
hypermutation have impaired gut homeostasis and compromised mucosal
defense. Nature immunology 2011; 12: 264-270. http://dx.doi.org/10.1038/ni.1991
- Kamada N, Nunez G. Regulation of the immune system
by the resident intestinal bacteria. Gastroenterology 2014; 146:
1477-1488. http://dx.doi.org/10.1053/j.gastro.2014.01.060
- Franchi
L, Kamada N, Nakamura Y, Burberry A, Kuffa P, Suzuki S, Shaw MH, Kim
YG, Nunez G. NLRC4-driven production of IL-1beta discriminates between
pathogenic and commensal bacteria and promotes host intestinal defense.
Nature immunology 2012; 13: 449-456. http://dx.doi.org/10.1038/ni.2263
- Hasegawa
M, Kamada N, Jiao Y, Liu MZ, Nunez G, Inohara N. Protective role of
commensals against Clostridium difficile infection via an
IL-1beta-mediated positive-feedback loop. Journal of immunology 2012;
189: 3085-3091. http://dx.doi.org/10.4049/jimmunol.1200821
- Luzza
F, Parrello T, Monteleone G, Sebkova L, Romano M, Zarrilli R, Imeneo M,
Pallone F. Up-regulation of IL-17 is associated with bioactive IL-8
expression in Helicobacter pylori-infected human gastric mucosa.
Journal of immunology 2000; 165: 5332-5337 http://dx.doi.org/10.4049/jimmunol.165.9.5332
- Ivanov,
II, Frutos Rde L, Manel N, Yoshinaga K, Rifkin DB, Sartor RB, Finlay
BB, Littman DR. Specific microbiota direct the differentiation of
IL-17-producing T-helper cells in the mucosa of the small intestine.
Cell host & microbe 2008; 4: 337-349. http://dx.doi.org/10.1016/j.chom.2008.09.009
- Gaboriau-Routhiau
V, Rakotobe S, Lecuyer E, Mulder I, Lan A, Bridonneau C, Rochet V, Pisi
A, De Paepe M, Brandi G, Eberl G, Snel J, Kelly D, Cerf-Bensussan N.
The key role of segmented filamentous bacteria in the coordinated
maturation of gut helper T cell responses. Immunity 2009; 31: 677-689. http://dx.doi.org/10.1016/j.immuni.2009.08.020
- Ivanov,
II, Atarashi K, Manel N, Brodie EL, Shima T, Karaoz U, Wei D, Goldfarb
KC, Santee CA, Lynch SV, Tanoue T, Imaoka A, Itoh K, Takeda K, Umesaki
Y, Honda K, Littman DR. Induction of intestinal Th17 cells by segmented
filamentous bacteria. Cell 2009; 139: 485-498. http://dx.doi.org/10.1016/j.cell.2009.09.033
- Lau
K, Benitez P, Ardissone A, Wilson TD, Collins EL, Lorca G, Li N, Sankar
D, Wasserfall C, Neu J, Atkinson MA, Shatz D, Triplett EW, Larkin J,
3rd. Inhibition of type 1 diabetes correlated to a Lactobacillus
johnsonii N6.2-mediated Th17 bias. Journal of immunology 2011; 186:
3538-3546. http://dx.doi.org/10.4049/jimmunol.1001864
- Macpherson
AJ, Geuking MB, McCoy KD. Homeland security: IgA immunity at the
frontiers of the body. Trends in immunology 2012; 33: 160-167. http://dx.doi.org/10.1016/j.it.2012.02.002
- Wesemann
DR, Portuguese AJ, Meyers RM, Gallagher MP, Cluff-Jones K, Magee JM,
Panchakshari RA, Rodig SJ, Kepler TB, Alt FW. Microbial colonization
influences early B-lineage development in the gut lamina propria.
Nature 2013; 501: 112-115.10.1038/nature12496 http://dx.doi.org/10.1038/nature12496
- Gordon
HA, Bruckner-Kardoss E, Wostmann BS. Aging in germ-free mice: life
tables and lesions observed at natural death. Journal of gerontology
1966; 21: 380-387 http://dx.doi.org/10.1093/geronj/21.3.380
PMid:5944800
- Hamada
H, Hiroi T, Nishiyama Y, Takahashi H, Masunaga Y, Hachimura S,
Kaminogawa S, Takahashi-Iwanaga H, Iwanaga T, Kiyono H, Yamamoto H,
Ishikawa H. Identification of multiple isolated lymphoid follicles on
the antimesenteric wall of the mouse small intestine. Journal of
immunology 2002; 168: 57-64 http://dx.doi.org/10.4049/jimmunol.168.1.57
- Bouskra
D, Brezillon C, Berard M, Werts C, Varona R, Boneca IG, Eberl G.
Lymphoid tissue genesis induced by commensals through NOD1 regulates
intestinal homeostasis. Nature 2008; 456: 507-510. http://dx.doi.org/10.1038/nature07450
- Fagarasan
S, Kawamoto S, Kanagawa O, Suzuki K. Adaptive immune regulation in the
gut: T cell-dependent and T cell-independent IgA synthesis. Annual
review of immunology 2010; 28: 243-273. http://dx.doi.org/10.1146/annurev-immunol-030409-101314
- Shroff
KE, Meslin K, Cebra JJ. Commensal enteric bacteria engender a
self-limiting humoral mucosal immune response while permanently
colonizing the gut. Infection and immunity 1995; 63:
3904-3913 PMid:7558298 PMCid:PMC173549
- Talham
GL, Jiang HQ, Bos NA, Cebra JJ. Segmented filamentous bacteria are
potent stimuli of a physiologically normal state of the murine gut
mucosal immune system. Infection and immunity 1999; 67: 1992-2000
PMid:10085047 PMCid:PMC96557
- Geuking
MB, Cahenzli J, Lawson MA, Ng DC, Slack E, Hapfelmeier S, McCoy KD,
Macpherson AJ. Intestinal bacterial colonization induces mutualistic
regulatory T cell responses. Immunity 2011; 34: 794-806. http://dx.doi.org/10.1016/j.immuni.2011.03.021
- Atarashi
K, Tanoue T, Shima T, Imaoka A, Kuwahara T, Momose Y, Cheng G, Yamasaki
S, Saito T, Ohba Y, Taniguchi T, Takeda K, Hori S, Ivanov, II, Umesaki
Y, Itoh K, Honda K. Induction of colonic regulatory T cells by
indigenous Clostridium species. Science 2011; 331: 337-341. http://dx.doi.org/10.1126/science.1198469
- Atarashi
K, Tanoue T, Oshima K, Suda W, Nagano Y, Nishikawa H, Fukuda S, Saito
T, Narushima S, Hase K, Kim S, Fritz JV, Wilmes P, Ueha S, Matsushima
K, Ohno H, Olle B, Sakaguchi S, Taniguchi T, Morita H, Hattori M, Honda
K. Treg induction by a rationally selected mixture of Clostridia
strains from the human microbiota. Nature 2013; 500: 232-236. http://dx.doi.org/10.1038/nature12331
- Dewhirst
FE, Chien CC, Paster BJ, Ericson RL, Orcutt RP, Schauer DB, Fox JG.
Phylogeny of the defined murine microbiota: altered Schaedler flora.
Applied and environmental microbiology 1999; 65: 3287-3292
PMid:10427008 PMCid:PMC91493
- Round
JL, Mazmanian SK. Inducible Foxp3+ regulatory T-cell development by a
commensal bacterium of the intestinal microbiota. Proceedings of the
National Academy of Sciences of the United States of America 2010; 107:
12204-12209. http://dx.doi.org/10.1073/pnas.0909122107
- Round
JL, Lee SM, Li J, Tran G, Jabri B, Chatila TA, Mazmanian SK. The
Toll-like receptor 2 pathway establishes colonization by a commensal of
the human microbiota. Science 2011; 332: 974-977. http://dx.doi.org/10.1126/science.1206095
- Smith
PM, Howitt MR, Panikov N, Michaud M, Gallini CA, Bohlooly YM, Glickman
JN, Garrett WS. The microbial metabolites, short-chain fatty acids,
regulate colonic Treg cell homeostasis. Science 2013; 341: 569-573. http://dx.doi.org/10.1126/science.1241165
- Furusawa
Y, Obata Y, Fukuda S, Endo TA, Nakato G, Takahashi D, Nakanishi Y,
Uetake C, Kato K, Kato T, Takahashi M, Fukuda NN, Murakami S, Miyauchi
E, Hino S, Atarashi K, Onawa S, Fujimura Y, Lockett T, Clarke JM,
Topping DL, Tomita M, Hori S, Ohara O, Morita T, Koseki H, Kikuchi J,
Honda K, Hase K, Ohno H. Commensal microbe-derived butyrate induces the
differentiation of colonic regulatory T cells. Nature 2013; 504:
446-450. http://dx.doi.org/10.1038/nature12721
- Arpaia
N, Campbell C, Fan X, Dikiy S, van der Veeken J, deRoos P, Liu H, Cross
JR, Pfeffer K, Coffer PJ, Rudensky AY. Metabolites produced by
commensal bacteria promote peripheral regulatory T-cell generation.
Nature 2013; 504: 451-455. http://dx.doi.org/10.1038/nature12726
- Le
Poul E, Loison C, Struyf S, Springael JY, Lannoy V, Decobecq ME,
Brezillon S, Dupriez V, Vassart G, Van Damme J, Parmentier M, Detheux
M. Functional characterization of human receptors for short chain fatty
acids and their role in polymorphonuclear cell activation. The Journal
of biological chemistry 2003; 278: 25481-25489. http://dx.doi.org/10.1074/jbc.M301403200
- Vinolo
MA, Rodrigues HG, Nachbar RT, Curi R. Regulation of inflammation by
short chain fatty acids. Nutrients 2011; 3: 858-876.10.3390/nu3100858 http://dx.doi.org/10.3390/nu3100858
- Coombes
JL, Siddiqui KR, Arancibia-Carcamo CV, Hall J, Sun CM, Belkaid Y,
Powrie F. A functionally specialized population of mucosal CD103+ DCs
induces Foxp3+ regulatory T cells via a TGF-beta and retinoic
acid-dependent mechanism. The Journal of experimental medicine 2007;
204: 1757-1764. http://dx.doi.org/10.1084/jem.20070590
- Sun
CM, Hall JA, Blank RB, Bouladoux N, Oukka M, Mora JR, Belkaid Y. Small
intestine lamina propria dendritic cells promote de novo generation of
Foxp3 T reg cells via retinoic acid. The Journal of experimental
medicine 2007; 204: 1775-1785. http://dx.doi.org/10.1084/jem.20070602
- Mucida
D, Park Y, Kim G, Turovskaya O, Scott I, Kronenberg M, Cheroutre H.
Reciprocal TH17 and regulatory T cell differentiation mediated by
retinoic acid. Science 2007; 317: 256-260. http://dx.doi.org/10.1126/science.1145697
- Welty
NE, Staley C, Ghilardi N, Sadowsky MJ, Igyarto BZ, Kaplan DH.
Intestinal lamina propria dendritic cells maintain T cell homeostasis
but do not affect commensalism. The Journal of experimental medicine
2013; 210: 2011-2024. http://dx.doi.org/10.1084/jem.20130728
- Singh
N, Gurav A, Sivaprakasam S, Brady E, Padia R, Shi H, Thangaraju M,
Prasad PD, Manicassamy S, Munn DH, Lee JR, Offermanns S, Ganapathy V.
Activation of Gpr109a, receptor for niacin and the commensal metabolite
butyrate, suppresses colonic inflammation and carcinogenesis. Immunity
2014; 40: 128-139. http://dx.doi.org/10.1016/j.immuni.2013.12.007
- Kim
SV, Xiang WV, Kwak C, Yang Y, Lin XW, Ota M, Sarpel U, Rifkin DB, Xu R,
Littman DR. GPR15-mediated homing controls immune homeostasis in the
large intestine mucosa. Science 2013; 340: 1456-1459.
http://dx.doi.org/10.1126/science.1237013
- Rossi M, Amaretti A, Raimondi S. Folate production
by probiotic bacteria. Nutrients 2011; 3: 118-134. http://dx.doi.org/10.3390/nu3010118
- Rivollier
A, He J, Kole A, Valatas V, Kelsall BL. Inflammation switches the
differentiation program of Ly6Chi monocytes from antiinflammatory
macrophages to inflammatory dendritic cells in the colon. The Journal
of experimental medicine 2012; 209: 139-155. http://dx.doi.org/10.1084/jem.20101387
- Storb
R, Prentice RL, Buckner CD, Clift RA, Appelbaum F, Deeg J, Doney K,
Hansen JA, Mason M, Sanders JE, Singer J, Sullivan KM, Witherspoon RP,
Thomas ED. Graft-versus-host disease and survival in patients with
aplastic anemia treated by marrow grafts from HLA-identical siblings.
Beneficial effect of a protective environment. The New England journal
of medicine 1983; 308: 302-307. http://dx.doi.org/10.1056/NEJM198302103080602
- Passweg
JR, Baldomero H, Bader P, Bonini C, Cesaro S, Dreger P, Duarte RF,
Dufour C, Falkenburg JH, Farge-Bancel D, Gennery A, Kroger N, Lanza F,
Nagler A, Sureda A, Mohty M, European Society for B, Marrow T.
Hematopoietic SCT in Europe 2013: recent trends in the use of
alternative donors showing more haploidentical donors but fewer cord
blood transplants. Bone marrow transplantation 2015; 50: 476-482. http://dx.doi.org/10.1038/bmt.2014.312
- Socie
G, Blazar BR. Acute graft-versus-host disease: from the bench to the
bedside. Blood 2009; 114: 4327-4336.10.1182/blood-2009-06-204669 http://dx.doi.org/10.1182/blood-2009-06-204669
- Biagi
E, Zama D, Nastasi C, Consolandi C, Fiori J, Rampelli S, Turroni S,
Centanni M, Severgnini M, Peano C, de Bellis G, Basaglia G, Gotti R,
Masetti R, Pession A, Brigidi P, Candela M. Gut microbiota trajectory
in pediatric patients undergoing hematopoietic SCT. Bone marrow
transplantation 2015; 50: 992-998.10.1038/bmt.2015.16 http://dx.doi.org/10.1038/bmt.2015.16
- Ferrara JL, Levine JE, Reddy P, Holler E.
Graft-versus-host disease. Lancet 2009; 373: 1550-1561. http://dx.doi.org/10.1016/S0140-6736(09)60237-3
- Shlomchik WD. Graft-versus-host disease. Nature
reviews Immunology 2007; 7: 340-352. http://dx.doi.org/10.1038/nri2000
- Heidegger
S, van den Brink MR, Haas T, Poeck H. The role of pattern-recognition
receptors in graft-versus-host disease and graft-versus-leukemia after
allogeneic stem cell transplantation. Frontiers in immunology 2014; 5:
337. http://dx.doi.org/10.3389/fimmu.2014.00337
- Welniak
LA, Blazar BR, Murphy WJ. Immunobiology of allogeneic hematopoietic
stem cell transplantation. Annual review of immunology 2007; 25:
139-170. http://dx.doi.org/10.1146/annurev.immunol.25.022106.141606
- Jones
JM, Wilson R, Bealmear PM. Mortality and gross pathology of secondary
disease in germfree mouse radiation chimeras. Radiation research 1971;
45: 577-588 http://dx.doi.org/10.2307/3573066
PMid:4396814
- van
Bekkum DW, Roodenburg J, Heidt PJ, van der Waaij D. Mitigation of
secondary disease of allogeneic mouse radiation chimeras by
modification of the intestinal microflora. Journal of the National
Cancer Institute 1974; 52: 401-404 PMid:4150164
- Taur
Y, Xavier JB, Lipuma L, Ubeda C, Goldberg J, Gobourne A, Lee YJ, Dubin
KA, Socci ND, Viale A, Perales MA, Jenq RR, van den Brink MR, Pamer EG.
Intestinal domination and the risk of bacteremia in patients undergoing
allogeneic hematopoietic stem cell transplantation. Clinical infectious
diseases: an official publication of the Infectious Diseases Society of
America 2012; 55: 905-914. http://dx.doi.org/10.1093/cid/cis580
- McDonald-Hyman
C, Turka LA, Blazar BR. Advances and challenges in immunotherapy for
solid organ and hematopoietic stem cell transplantation. Science
translational medicine 2015; 7: 280rv282. http://dx.doi.org/10.1126/scitranslmed.aaa6853
- Jenq
RR, Ubeda C, Taur Y, Menezes CC, Khanin R, Dudakov JA, Liu C, West ML,
Singer NV, Equinda MJ, Gobourne A, Lipuma L, Young LF, Smith OM, Ghosh
A, Hanash AM, Goldberg JD, Aoyama K, Blazar BR, Pamer EG, van den Brink
MR. Regulation of intestinal inflammation by microbiota following
allogeneic bone marrow transplantation. The Journal of experimental
medicine 2012; 209: 903-911. http://dx.doi.org/10.1084/jem.20112408
- Taur
Y, Jenq RR, Perales MA, Littmann ER, Morjaria S, Ling L, No D, Gobourne
A, Viale A, Dahi PB, Ponce DM, Barker JN, Giralt S, van den Brink M,
Pamer EG. The effects of intestinal tract bacterial diversity on
mortality following allogeneic hematopoietic stem cell transplantation.
Blood 2014; 124: 1174-1182. http://dx.doi.org/10.1182/blood-2014-02-554725
- Jenq
RR, Taur Y, Devlin SM, Ponce DM, Goldberg JD, Ahr KF, Littmann ER, Ling
L, Gobourne AC, Miller LC, Docampo MD, Peled JU, Arpaia N, Cross JR,
Peets TK, Lumish MA, Shono Y, Dudakov JA, Poeck H, Hanash AM, Barker
JN, Perales MA, Giralt SA, Pamer EG, van den Brink MR. Intestinal
Blautia Is Associated with Reduced Death from Graft-versus-Host
Disease. Biology of blood and marrow transplantatio: journal of the
American Society for Blood and Marrow Transplantation 2015; 21:
1373-1383. http://dx.doi.org/10.1016/j.bbmt.2015.04.016
- Holler
E, Butzhammer P, Schmid K, Hundsrucker C, Koestler J, Peter K, Zhu W,
Sporrer D, Hehlgans T, Kreutz M, Holler B, Wolff D, Edinger M,
Andreesen R, Levine JE, Ferrara JL, Gessner A, Spang R, Oefner PJ.
Metagenomic analysis of the stool microbiome in patients receiving
allogeneic stem cell transplantation: loss of diversity is associated
with use of systemic antibiotics and more pronounced in
gastrointestinal graft-versus-host disease. Biology of blood and marrow
transplantation: journal of the American Society for Blood and Marrow
Transplantation 2014; 20: 640-645.10.1016/j.bbmt. http://dx.doi.org/2014.01.030
- Chiusolo
P, Metafuni E, Paroni Sterbini F, Giammarco S, Masucci L, Leone G, Sica
S. Gut Microbiome Changes after Stem Cell Transplantation. Blood 2015;
126: 1953
- Eriguchi
Y, Takashima S, Oka H, Shimoji S, Nakamura K, Uryu H, Shimoda S,
Iwasaki H, Shimono N, Ayabe T, Akashi K, Teshima T. Graft-versus-host
disease disrupts intestinal microbial ecology by inhibiting Paneth cell
production of alpha-defensins. Blood 2012; 120: 223-231. http://dx.doi.org/10.1182/blood-2011-12-401166
- Levine
JE, Huber E, Hammer ST, Harris AC, Greenson JK, Braun TM, Ferrara JL,
Holler E. Low Paneth cell numbers at onset of gastrointestinal
graft-versus-host disease identify patients at high risk for nonrelapse
mortality. Blood 2013; 122: 1505-1509. http://dx.doi.org/10.1182/blood-2013-02-485813
- Ferrara
JL, Harris AC, Greenson JK, Braun TM, Holler E, Teshima T, Levine JE,
Choi SW, Huber E, Landfried K, Akashi K, Vander Lugt M, Reddy P, Chin
A, Zhang Q, Hanash S, Paczesny S. Regenerating islet-derived 3-alpha is
a biomarker of gastrointestinal graft-versus-host disease. Blood 2011;
118: 6702-6708. http://dx.doi.org/10.1182/blood-2011-08-375006
- Rayes
A, Morrow AL, Payton LR, Lake KE, Lane A, Davies SM. A Genetic Modifier
of the Gut Microbiome Influences the Risk of Graft-versus-Host Disease
and Bacteremia After Hematopoietic Stem Cell Transplantation. Biology
of blood and marrow transplantation: journal of the American Society
for Blood and Marrow Transplantation 2015, http://dx.doi.org/10.1016/j.bbmt.2015.11.017
- Rouquier
S, Lowe JB, Kelly RJ, Fertitta AL, Lennon GG, Giorgi D. Molecular
cloning of a human genomic region containing the H blood group
alpha(1,2)fucosyltransferase gene and two H locus-related DNA
restriction fragments. Isolation of a candidate for the human Secretor
blood group locus. The Journal of biological chemistry 1995; 270:
4632-4639 http://dx.doi.org/10.1074/jbc.270.9.4632
PMid:7876234
- Tong
M, McHardy I, Ruegger P, Goudarzi M, Kashyap PC, Haritunians T, Li X,
Graeber TG, Schwager E, Huttenhower C, Fornace AJ, Jr., Sonnenburg JL,
McGovern DP, Borneman J, Braun J. Reprograming of gut microbiome energy
metabolism by the FUT2 Crohn's disease risk polymorphism. The ISME
journal 2014; 8: 2193-2206. http://dx.doi.org/10.1038/ismej.2014.64
- McGovern
DP, Jones MR, Taylor KD, Marciante K, Yan X, Dubinsky M, Ippoliti A,
Vasiliauskas E, Berel D, Derkowski C, Dutridge D, Fleshner P, Shih DQ,
Melmed G, Mengesha E, King L, Pressman S, Haritunians T, Guo X, Targan
SR, Rotter JI, International IBDGC. Fucosyltransferase 2 (FUT2)
non-secretor status is associated with Crohn's disease. Human molecular
genetics 2010; 19: 3468-3476. http://dx.doi.org/10.1093/hmg/ddq248
- Vossen
JM, Heidt PJ, van den Berg H, Gerritsen EJ, Hermans J, Dooren LJ.
Prevention of infection and graft-versus-host disease by suppression of
intestinal microflora in children treated with allogeneic bone marrow
transplantation. European journal of clinical microbiology &
infectious diseases: official publication of the European Society of
Clinical Microbiology 1990; 9: 14-23 http://dx.doi.org/10.1007/BF01969527
- Vossen
JM, Guiot HF, Lankester AC, Vossen AC, Bredius RG, Wolterbeek R, Bakker
HD, Heidt PJ. Complete suppression of the gut microbiome prevents acute
graft-versus-host disease following allogeneic bone marrow
transplantation. PloS one 2014; 9: e105706. http://dx.doi.org/10.1371/journal.pone.0105706
- Docampo
MD, Auletta JJ, Jenq RR. Emerging Influence of the Intestinal
Microbiota during Allogeneic Hematopoietic Cell Transplantation:
Control the Gut and the Body Will Follow. Biology of blood and marrow
transplantation: journal of the American Society for Blood and Marrow
Transplantation 2015; 21: 1360-1366. http://dx.doi.org/10.1016/j.bbmt.2015.02.016
- Murphy S, Nguyen VH. Role of gut microbiota in
graft-versus-host disease. Leukemia & lymphoma 2011; 52: 1844-1856.
http://dx.doi.org/10.3109/10428194.2011.580476
- Lozupone
CA, Stombaugh JI, Gordon JI, Jansson JK, Knight R. Diversity, stability
and resilience of the human gut microbiota. Nature 2012; 489: 220-230. http://dx.doi.org/10.1038/nature11550
- Gerbitz
A, Schultz M, Wilke A, Linde HJ, Scholmerich J, Andreesen R, Holler E.
Probiotic effects on experimental graft-versus-host disease: let them
eat yogurt. Blood 2004; 103: 4365-4367. http://dx.doi.org/10.1182/blood-2003-11-3769
- Schrezenmeir
J, de Vrese M. Probiotics, prebiotics, and synbiotics--approaching a
definition. The American journal of clinical nutrition 2001; 73:
361S-364S PMid:11157342
- Hennet T, Weiss A, Borsig L. Decoding breast milk
oligosaccharides. Swiss medical weekly 2014; 144: w13927. http://dx.doi.org/10.4414/smw.2014.13927
- Yu
ZT, Chen C, Newburg DS. Utilization of major fucosylated and sialylated
human milk oligosaccharides by isolated human gut microbes.
Glycobiology 2013; 23: 1281-1292. http://dx.doi.org/10.1093/glycob/cwt065
- Newburg
DS, He Y. Neonatal Gut Microbiota and Human Milk Glycans Cooperate to
Attenuate Infection and Inflammation. Clinical obstetrics and
gynecology 2015; 58: 814-826. http://dx.doi.org/10.1097/GRF.0000000000000156
- He
Y, Liu S, Kling DE, Leone S, Lawlor NT, Huang Y, Feinberg SB, Hill DR,
Newburg DS. The human milk oligosaccharide 2'-fucosyllactose modulates
CD14 expression in human enterocytes, thereby attenuating LPS-induced
inflammation. Gut 2016; 65: 33-46. http://dx.doi.org/10.1136/gutjnl-2014-307544
- Newburg
DS, Ko JS, Leone S, Nanthakumar NN. Human Milk Oligosaccharides and
Synthetic Galactosyloligosaccharides Contain 3'-, 4-, and
6'-Galactosyllactose and Attenuate Inflammation in Human T84, NCM-460,
and H4 Cells and Intestinal Tissue Ex Vivo. The Journal of nutrition
2016; 146: 358-367. http://dx.doi.org/10.3945/jn.115.220749
- Ortega-Gonzalez
M, Ocon B, Romero-Calvo I, Anzola A, Guadix E, Zarzuelo A, Suarez MD,
Sanchez de Medina F, Martinez-Augustin O. Nondigestible
oligosaccharides exert nonprebiotic effects on intestinal epithelial
cells enhancing the immune response via activation of TLR4-NFkappaB.
Molecular nutrition & food research 2014; 58: 384-393. http://dx.doi.org/10.1002/mnfr.201300296
- Neemann
K, Eichele DD, Smith PW, Bociek R, Akhtari M, Freifeld A. Fecal
microbiota transplantation for fulminant Clostridium difficile
infection in an allogeneic stem cell transplant patient. Transplant
infectious disease: an official journal of the Transplantation Society
2012; 14: E161-165. http://dx.doi.org/10.1111/tid.12017
- de
Castro CG, Jr., Ganc AJ, Ganc RL, Petrolli MS, Hamerschlack N. Fecal
microbiota transplant after hematopoietic SCT: report of a successful
case. Bone marrow transplantation 2015; 50: 145. http://dx.doi.org/10.1038/bmt.2014.212
- Bilinski
J, Robak K, Peric Z, Marchel H, Karakulska-Prystupiuk E, Halaburda K,
Rusicka P, Swoboda-Kopec E, Wroblewska M, Wiktor-Jedrzejczak W, Basak
GW. Impact of Gut Colonization by Antibiotic-Resistant Bacteria on the
Outcomes of Allogeneic Hematopoietic Stem Cell Transplantation: A
Retrospective, Single-Center Study. Biology of blood and marrow
transplantation: journal of the American Society for Blood and Marrow
Transplantation 2016, DOI:
10.1016/j.bbmt.2016.02.009.10.1016/j.bbmt.2016.02.009
- Bilinski
J, Grzesiowski P, Muszynski J, Wroblewska M, Madry K, Robak K,
Dzieciatkowski T, Wiktor-Jedrzejczak W, Basak GW. Fecal Microbiota
Transplantation Inhibits Multidrug-Resistant Gut Pathogens: Preliminary
Report Performed in an Immunocompromised Host. Archivum immunologiae et
therapiae experimentalis 2016. http://dx.doi.org/10.1007/s00005-016-0387-9