Mervat A M Youssef1, Ebtisam Shawky Ahmed2, Dalia Tarik Kamal3, Khalid I Elsayh1, Mai A Abdelfattah1, Hyam Hassan Mahran1 and, Mostafa M Embaby1.
1 Children Hospital, Hematology Unit, Faculty of Medicine, Assiut University, Assiut, Egypt.
2 Clinical Pathology Department- Faculty of Medicine - New Valley University.
3 Clinical Pathology Department - Faculty of Medicine - Assiut University.
Correspondence to: Dr.
Mervat A. M. Youssef, MD, Children's Hospital, Hematology Unit, Faculty
of Medicine, Assiut-University, Egypt Address: Egypt,
Assiut University, Faculty of Medicine, Children's Hospital. Telephone
number: +2088201142606221. Fax number: 0882368371. Email:
mamuosif2000@aun.edu.eg. ORCID: 0000-0002-9054-0662
Published: March 01, 2024
Received: August 22, 2023
Accepted: February 07, 2024
Mediterr J Hematol Infect Dis 2024, 16(1): e2024034 DOI
10.4084/MJHID.2024.034
This is an Open Access article distributed
under the terms of the Creative Commons Attribution License
(https://creativecommons.org/licenses/by-nc/4.0),
which permits unrestricted use, distribution, and reproduction in any
medium, provided the original work is properly cited.
|
Abstract
Background: Viral infections can cause direct and indirect damage to hematopoietic stem cells. The
objectives of this study were to identify the frequency and severity of
aplastic anemia in children infected with severe acute respiratory
syndrome coronavirus 2 (SARS-CoV-2) as well as recognize the response
to treatment. Methodology:
13 children with newly diagnosed severe aplastic anemia were enrolled
in this prospective clinical trial. Blood samples were obtained from
all patients to detect SARS-CoV-2 antibodies, and nasopharyngeal swabs
were collected for reverse-transcription Polymerase Chain Reaction to
detect SARS-CoV-2 viruses. According to the laboratory
results, patients were classified as having SARS-CoV-2 positive
antibodies and SARS-CoV-2 negative antibodies. Both groups
received combined cyclosporine (CsA) + Eltrombopag (E-PAG). The
hematological response, either complete response (CR) or partial
response (PR), no response (NR), and overall response (OR) rates of
combined E-PAG + CsA treatment after 6 months were evaluated. Results:
Four children were recognized to have aplastic anemia and SARS-CoV-2
positive antibodies. Two patients fulfilled the hematological criteria
for CR and no longer required transfusion of packed red blood cells
(PRBCs) or platelets, and one had PR and was still PRBC
transfusion-dependent but no longer required platelet transfusion. The
remaining patient showed NR, and he had died before reaching the top of
the HSCT waiting list. Moreover, six patients in the SARS-CoV-2
negative antibodies group had CR, while three patients had PR. The
difference in ANC, Hg, and platelet counts between both groups was not
significant. Conclusion:
The SARS-CoV-2 virus is added to several viral infections known to be
implicated in the pathogenesis of aplastic anemia. Studies are needed
to establish a definitive association and determine whether the
response of bone marrow failure to standard therapy differs from that
of idiopathic cases.
|
Introduction
Acquired aplastic anemia (AAA) in childhood is a serious disorder characterized by pancytopenia and hypocellular bone marrow.[1] It
is a rare disorder with an incidence of about 2 per 1,000,000 children
per year in North America and Europe and 2–3 fold higher in Asia with
an equal male-to-female ratio.[2]
It has to be differentiated from inherited bone marrow failure syndromes (IBMFS), which are more common in children.[3]
The
pathophysiology of AAA is unknown; it has been suggested to be
immune-mediated. Several studies have verified the increased cytokine
expression, low CD4 T regulatory cells, oligoclonal CD8 cytotoxic T
cells, and expansion of specific CD4 cell subsets in the bone marrow
(BM).[4-6]
Viral infections can cause direct and
indirect damage to hematopoietic stem cells (HSCs). King and Goodell
demonstrated four different mechanisms by which viruses can alter HSC
biology.[7] Two mechanisms act via direct effects on
HSCs, including direct infection or direct recognition of a pathogen.
Two indirect mechanisms act through pro-inflammatory cytokines released
by other cells or through changes in the BM microenvironment.
Viral
infections that directly altered the HSCs include Epstein–Barr virus
(EBV), varicella-zoster virus (VZV), cytomegalovirus (CMV), human
immunodeficiency virus (HIV), human herpes virus 6
(HHV-6), hepatitis A and C viruses (HAV and HCV), dengue and
parvovirus B19.[8-11]
Indirect damage of
the HSCs resulting from acute or chronic viral infection has
often been attributed to the immune response against viruses, with IFNγ
and CD8+ T cells having a key role. The hematopoietic cells are the
target of oligoclonal CD8+ T cells, which produce IFNγ and TNFα and
cause its death. Otherwise, constant secretion of these
pro-inflammatory cytokines can also deplete HSCs, thus leading to
aplastic anemia.[12] Nevertheless, bone marrow
pathologies are rare and frequently related to changes in gene
regulation of cytokines, effector, and MHC molecules, which suggest a
genetic basis for abnormal T cell activation in BM failure.[11,13]
AAA is presenting clinically with easy bruising or petechiae,
epistaxis, and menorrhagia in postmenarchal girls due to
thrombocytopenia. Anemia may manifest as pallor and fatigue, while
neutropenia may predispose to infections.[14]
Severe
acute respiratory syndrome coronavirus 2 (SARS-CoV-2) is a novel
coronavirus . It is named COVID-19 and infected millions of people
worldwide since its outbreak in 2019.[15]
It is
a systemic disease and classically presents with flu-like systemic
and/or respiratory symptoms. Severe cases presented with acute
respiratory distress syndrome (ARDS) and Multi-organ failure,
which is the major cause of COVID-19-related death.[16] Moreover, one-third of patients with COVID-19 are asymptomatic.[17]
SARS-CoV-2 infection begins by sticking the viral surface spike
glycoprotein (S protein) to the angiotensin-converting enzyme-2
(ACE2) for initiation of cell entry. Also, it downregulates cell
surface ACE2 expression, leading to loss of the enzyme mediate
protective function.[18] The expression of ACE2 has been stated in a variety of cells, including HSCs.[19]
Several
researchers have verified the profound effect of COVID-19 disease on
the hematopoiesis system either by direct invasion of HSCs by
SARS-CoV-2, which may explain the mechanism of hypoxia or
hyper-inflammation and cytokine release, which might be related to
activation and proliferative of the hematopoietic system.[20,21]
All this leads to significant changes in the hematopoietic system,
including stress erythropoiesis, lymphopenia, neutrophilia, and
thrombocytopenia.[20,21]
To the best of our
knowledge, no study has been piloted to confirm the existence of
aplastic anemia in children with COVID-19 infection. Hence, the
objectives of this study were to identify the frequency and severity of
aplastic anemia in COVID-19-infected children and how it is related to
the severity of infection, as well as recognition of the response to
treatment.
Materials and
Method
This
study was a prospective, single-center clinical trial conducted
at Assiut University Children's Hospital in Egypt. It enrolled all
children with newly diagnosed aplastic anemia admitted to the clinical
hematology unit between Jun 2021 and December 2022. All enrolled children fulfilled the eligibility criteria[22] and met the modified Camitta criteria for severe aplastic anemia (SAA).[23]
According to these criteria, a diagnosis of SAA may be made if bone
marrow cellularity is <25% and/or at least two of the following
criteria are met: (i) the absolute neutrophil count is below 0.5 × 109/L, (ii) the platelet count is below 20 × 109/L, (iii) the reticulocyte count is below 20 × 109/L.
The
exclusion criteria were inherited bone marrow failure, myelodysplasia,
underproduction anemias secondary to B12, folate or iron deficiency, or
with other reversible causes.
All patients were subjected to a
detailed history regarding drug intake, radiation exposure, and recent
history of infections. All patients subjected to complete medical
history, physical examination, and laboratory evaluations included,
including a complete blood count (CBC) with differential, serum
chemistry, bone marrow aspiration and biopsy, viral serology,
immunological tests, flow cytometric tests, a diepoxybutane clastogenic
stress assay, and HLA typing. All patients were assessed for an
inherited bone marrow failure syndrome, including chromosomal breakage
examination for Fanconi anemia.
Blood samples were obtained from
all patients to detect SARS-CoV-2 antibodies, and nasopharyngeal swabs
were collected for reverse-transcription Polymerase Chain Reaction
(RT-PCR) to detect SARS-CoV-2 viruses.
The study was
permitted by Assiut University's Ethical Committee for Clinical
Research, and informed consent was obtained from the guardians of trial
participants before the study.
PCR.
All patients were subjected to PCR-RNA for SARS-CoV-2 (COBAS6800,
Roche, India Qiagen, Germany) for use on the cobas® 6800 System.
Nasopharyngeal and oropharyngeal swab samples were collected on 0.9%
physiological saline. Selective amplification of target nucleic acid
from the sample was achieved using specific forward and reverse primers
for ORF1 a/b non-structural region that is unique to SARS-CoV-2. The
pan-Sarbecovirus detection sets also detected the SARS-CoV-2 virus.
Amplification of RNA Internal Control was achieved using
non-competitive sequence-specific forward and reverse primers, which
have no homology with the coronavirus genome. DNA polymerase enzyme was
used for amplification.
Detection of antibodies to SARS-CoV-2.
A three ml blood sample was obtained from all participants on gel and
clot activator tubes for separation of sera for SARS-CoV-2 antibody
testing. Detection of SARS CoV 2 total antibody was performed by
Elecsys Anti SARS CoV 2 kit Lot No. 49546401 (Germany)
supplied by Roche based on electrochemiluminescence immunoassay "ECLIA"
using cobas e 411 immunoassay analyzers.
Treatment plan.
According to the PCR and antibodies to SARS-CoV-2 detection results,
patients were categorized into two groups: SARS-CoV-2 positive
antibodies and SARS-CoV-2 negative antibodies. Because of the long list
of patients in need of hematopoietic stem cell transplantation (HSCT)
in our country and the anti-thymocyte globulin (ATG) is unavailable for
economic reasons, both groups received combined cyclosporine (CsA)
+ Eltrombopag (E-PAG). The initial oral dose of E-PAG was 50 mg
once daily. The dose was escalated by 25 mg every two weeks in all
patients and then maintained at the maximum dose when it was reached.
The maximum dose was 150 mg.[25] Adjustments and reductions of the E-PAG dose were made where necessary based on the pharmacokinetic data for ITP.[24] Oral CsA was initiated at 5–10 mg/kg/day, and the dose was adjusted to maintain trough levels of 170–270 ng/ml.[24]
Supportive therapy was allowed for both groups during the study when
essential. It included granulocyte colony-stimulating factor (G-CSF),
iron chelation, or platelet transfusion (if the count
was <10,000/μL with an apparent bleeding tendency or
<20,000/μL with fever) and red blood cell (RBC) transfusion (if
hemoglobin was <7 g/dL or in the presence of significant symptoms,
such as exertional dyspnea or anemic heart failure). The hematological
response, either complete response (CR)or partial response (PR), no
response (NR), and overall response (OR) rates of combined E-PAG + CsA
treatment after 6 months were evaluated, using the standard guidelines
for the diagnosis and treatment of pediatric SAA.[25]
Response criteria.
A hematological response was defined as a platelet count increase of at
least 20,000/μL and/or platelet transfusion independence for a minimum
of eight weeks, a hemoglobin level increase of at least 1.5 g/L or a
reduction in the number of PRBCs units transfused by at least four for
eight consecutive weeks (compared with transfusion requirements during
the eight weeks preceding study treatment onset) and an increase of
absolute neutrophil count (ANC) of >500/μL in patients with a
pre-treatment count <500/μl. A PR was defined as a blood count no
longer meeting the Camitta criteria[22] for SAA and
no transfusion dependence for platelets or red blood cells. A CR was
defined as Hb levels of ≥100 g/l, a platelet count ≥100 × 109/L, ANC of ≥1 × 109/L, and transfusion and growth factor independence. Overall response rates included all PR and CR within each group.
Statistics.
Statistical Package for Social Science version 20. A T-test calculator
for 2 Independent Means was used to detect the statistical differences
between both groups. Descriptive statistics were expressed as
frequencies and percentages for categorical data. Fisher Exact Test was
used to detect the statistical differences in categorical variables. Data
represented as means ±SD. The probability value of <0.05 is
considered statistically significant.
Results
Thirteen
patients (6 boys and 7 girls) fulfilled the inclusion criteria.
The age at presentation ranged from 4.4 to 17.7 years (median 9.3
years) (Table 1).
Four
children (30.7%) without prior hematologic diseases or SARS-CoV-2
vaccination were recognized to have aplastic anemia and SARS-CoV-2
positive antibodies (Table 2).
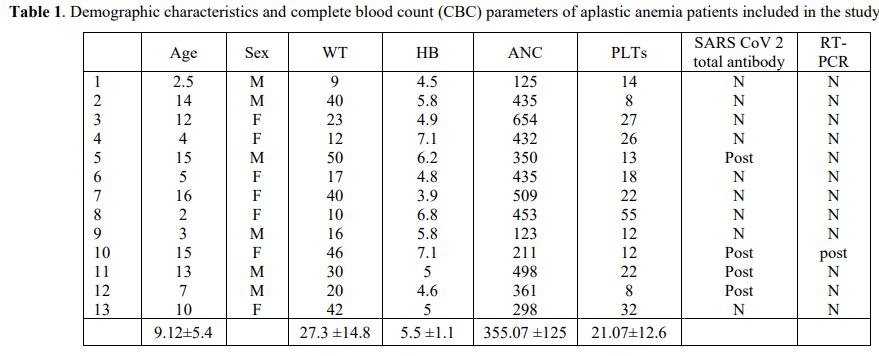 |
Table 1. Demographic characteristics and complete blood count (CBC) parameters of aplastic anemia patients included in the study |
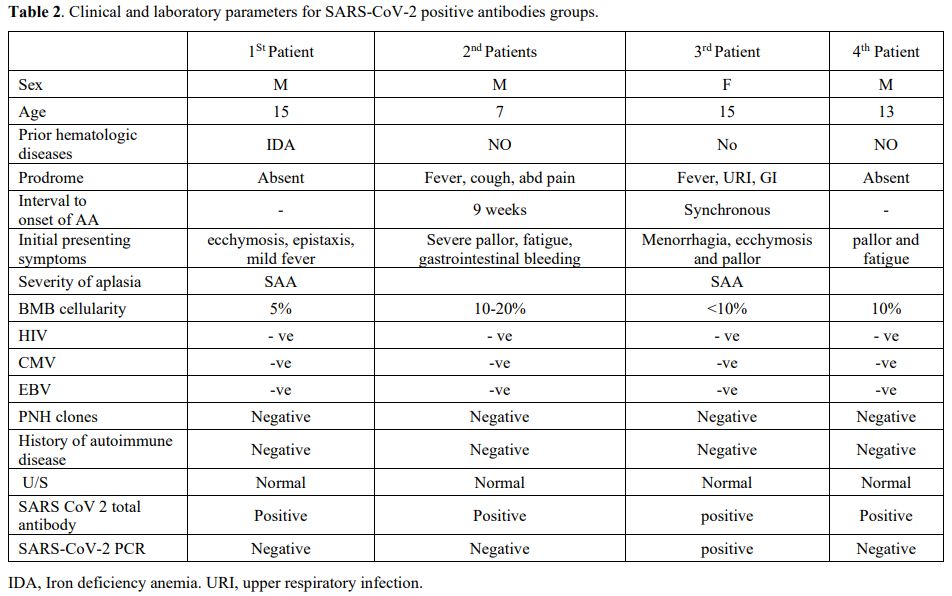 |
Table 2. Clinical and laboratory parameters for SARS-CoV-2 positive antibodies groups.
|
The
first patient was a 15-year-old boy who presented with ecchymosis,
epistaxis, and mild fever and was found to have pancytopenia. He did
not have any prodromal symptoms before the onset of pancytopenia. His
workup showed negative SARS-CoV-2 PCR and negative
hepatitis, cytomegalovirus (CMV), Epstein-Barr virus (EBV), HIV,
and PNH panel. Additionally, no nutritional deficiencies were
found. SARS-CoV-2 total antibodies were the only test positive test in
this patient. BM biopsy revealed severe hypoplasia (5%).
The
second was a 7-year-old boy; he had a high-grade fever, sore throat,
cough, and abdominal pain nine weeks before the onset of
pancytopenia. This patient presented to the emergency unit with severe
pallor, fatigue, and gastrointestinal bleeding, which necessitated
urgent transfusion of PRBCS and platelets. CBC revealed
pancytopenia, and other investigations (liver function, renal function,
abdominal ultrasound, CRP, ESR, were unremarkable. A bone marrow biopsy
verified hypocellularity (10%-20%) with all lineage without signs of
malignancy or megaloblastic changes (Table 2).
The
third patient was a 15-year-old girl. She had a fever, chest infection,
and mild gastroenteritis for 3 days, followed by massive menorrhagia,
ecchymosis, and pallor. She had not experienced a similar attack
previously. She had Synchronous pancytopenia at the time of diagnosis
of SARS-CoV-2 infection by positive PCR testing and positive
total immunoglobulins. She was diagnosed with SAA according to the
results of the bone marrow biopsy (< 10% cellularity).
The
fourth patient was a 13-year-old male with absent prodromal symptoms
before cytopenia, but he had a positive family history of SARS-CoV-2
infection. He presented with marked pallor and fatigue. Bone marrow
biopsy results confirmed the diagnosis of severe aplastic anemia
(<10% cellularity).
No PNH clone or
evidence of inherited bone marrow failure syndrome was recognized in
all SARS-CoV-2 antibodies-positive children. Additionally,
all were negative for other viral testing.
Transfusion rate and Treatment results after 6 months. The hematological response of the SARS-CoV-2 positive antibodies after six months of treatment was recorded in Table 3. Two patients (50%) fulfilled the hematological criteria[26]
for CR and no longer required transfusion of packed red blood cells
(PRBCs) or platelets. One (25%) more patients had a partial
response (PR) and were still PRBC transfusion-dependent but no longer
required platelet transfusion. The remaining patient (25%) did not meet
any of the response criteria, and he had died before reaching the top
of the HSCT waiting list.
Moreover, six patients in the
SARS-CoV-2 negative antibodies group had CR (66.6%), while three
patients (333.3%) had PR. The difference in ANC, Hg, and platelet
counts between both groups was not significant (Table 4).
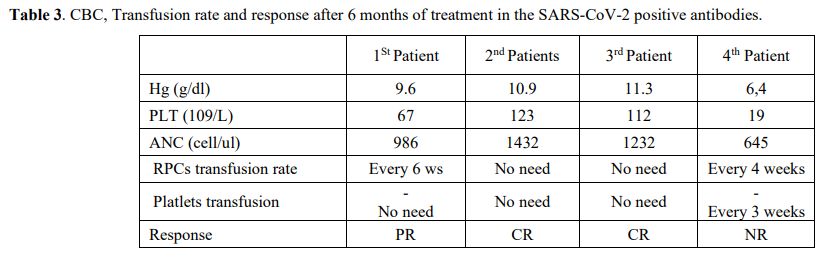 |
Table 3. CBC, Transfusion rate and response after 6 months of treatment in the SARS-CoV-2 positive antibodies |
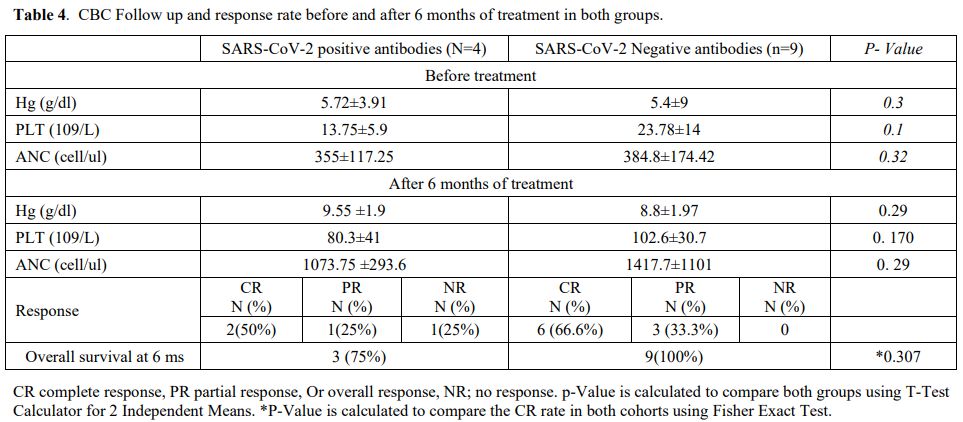 |
Table 4. CBC Follow up and response rate before and after 6 months of treatment in both groups.
|
Discussion
Acquired
aplastic anemia is a rare disorder characterized by damage to
progenitor cells caused by chemicals, drugs, ionizing radiation, viral
infections, or autoimmune destruction. The majority of aplastic anemia
cases (70-80%) are idiopathic, with viral infections representing only
a small portion of these cases.[27,28]
Among the
13 cases of newly diagnosed aplastic anemia admitted to our unit within
18 months duration, we report 4 cases associated with COVID-19
infection. A recent large case series of new-onset AA in adults
associated with preceding SARS-CoV-2 infection was reported.[29]
In
another case series conducted with Avenoso et al., they reported that
three adults were diagnosed with AA a few weeks after SARS-CoV-2
infection.[30] Hock et al. also reported severe aplastic anemia in a 21-year-old man infected with SARS-CoV-2.[30,31]
Moreover,
Rohini Chakravarthy et al. confirmed the diagnosis of SAA in a
12-year-old girl and an 18-year-old male. Both patients received
anti‐thymoglobulin (ATG) and cyclosporine and became transfusion
independent.[32]
Viral-induced bone marrow
suppression is well established, and different mechanisms have been
proposed for its occurrence, including directly influencing the
replication of hematopoietic stem and progenitor cells (HSPCs) or
indirectly by inducing different patterns of cytokines and chemokines
such as IL-1ß, IL-6, TNF-α, and INF-γ, which cause Fas-mediated
apoptosis. Direct destruction of HPSC by viruses has been observed as
well.[33]
The SARS-CoV-2 infection has a
significant impact on the host's immune system. Variable degrees of
lymphopenia (CD3 + T, CD4 + T, or CD8 + T cells) are observed in mild
to moderate COVID-19 phenotypes; however, the decline in T lymphocyte
count considerably worsens in severe cases.[33-35]
In most cases, pancytopenia is mild and transient and does not necessitate bone marrow examination.[36]
In the present study, one patient did not show any response and was dependent on transfusion until death. Ranjima et al.[36]
reported that a 4-year-old girl developed aplastic anemia after COVID,
which did not respond satisfactorily to treatment, and became a
candidate for bone marrow transplantation. Fatemeh et al.[37]
described a 16-year-old girl who developed severe aplastic anemia with
COVID infection and did not respond well to treatment, even though she
received supportive treatment and immunosuppression.
Poor response
to treatment is predicted by low CD8 + T and B cell numbers and a high
CD4/CD8 ratio. In the phenotypes of serious diseases, the production of
interferon-gamma (IFN-γ) by CD4 + T lymphocytes is also diminished by.[38,39]
Another
rare outcome of SARS-CoV-2 in children associated with abnormal innate
and adaptive immune responses is known as multisystem inflammatory
syndrome (MIS), which is characterized by a cytokine storm.[40,41]
Even though the effects of SARS-CoV-2 infection on host immune
responses are well documented, bone marrow-induced aplasia (BM) is less
well understood. The exact mechanism behind COVID-19 could induce bone
marrow aplasia has not yet been fully explained, but it is
multifactorial.
Numerous cases of severe central pancytopenia related to COVID-19 have been reported.[42-44]
While most cases were transient and did not require bone marrow
biopsies, the four cases in our study, with observation, did not
demonstrate spontaneous resolution of peripheral cytopenias and marrow
hypocellularity, arguing against a diagnosis of viral myelosuppression.
Some other case series have shown that SARS-CoV2 may cause bone marrow
failure requiring immunosuppressive therapy (IST) or even hematopoietic
stem cell transplantation (HSCT).[30-32]
The
role of autoimmune cytotoxic T-cell-related hematopoietic cell
destruction causing aplastic anemia in COVID-19 patients is still
undiscovered. For COVID-19 to trigger immune-mediated bone marrow
failure, infection should precede pancytopenia by weeks to months. Half
of our patients had an unknown duration between the onset of infection
and pancytopenia (Patients 1 and 4). These patients had negative PCR
but positive SARS-CoV-2 antibodies, suggesting distant infection. One
of the remaining two patients had an infection 9 weeks before
pancytopenia, while the last one (Patient 3) had pancytopenia 3 days
after the onset of COVID-19 symptoms.
Regarding management and
follow-up, all of our patients were treated with immunosuppression
(Cyclosporin) plus Eltrombopag with variable response. Two patients had
variable responses. Two other cases (Patients 2 and 3) showed a
complete response, One patient showed a partial response (Patient 1),
and one did not respond at all and died (Patient 4).
The
response to Cyclosporin and whether the addition of eltrombopag
improves the treatment results in these patients are also issues. It
has been assumed that eltrombopag can potentiate the effect of
thrombopoietin (TPO) in vivo, overcoming the suppressive effect of
IFN-γ upon TPO signaling in hematopoietic stem cells.[45]
Overall,
the epidemiological data and prior SARS-CoV-2 infection estimated to
have preceded the development of pancytopenia by weeks to months
support the theory that SARS-CoV-2 may be causally associated with AA.
Besides, regardless of disease phenotype (even in asymptomatic cases),
SARS-CoV-2 infection could be associated with severe aplastic anemia.
This study does not establish a mechanistic link between COVID-19
infection and marrow failure; however, the clinical course and response
to Cyclosporin have led to our hypothesis that this novel coronavirus
may mediate an immune response or, less likely, a direct marrow
toxicity that contributes to the pathogenesis of SAA.
Conclusions
We
report one of the largest case series to date of the new
onset of SAA in paediatrics, presumably associated with preceding
SARS-CoV-2 infection and their clinical outcomes. Considering the
results of this study, the virus is added to several viral infections
known to be implicated in the pathogenesis of aplastic anaemia. More
studies are needed to establish a definitive association and determine
whether the natural history and response of bone marrow failure to
standard therapy differ from that of idiopathic cases.
References
- Bacigalupo A, Hows J, Gluckman E, et al. Bone
marrow transplantation (BMT) versus immunosuppression for the treatment
of severe aplastic anaemia (SAA): a report of the EBMT SAA working
party. Br J Haematol. 1988;70:177-182. https://doi.org/10.1111/j.1365-2141.1988.tb02460.x PMid:3056497
- Montane
E, Ibanez L, Vidal X, et al. Epidemiology of aplastic anemia: a
prospective multicenter study. Haematologica. 2008;93:518-523. https://doi.org/10.3324/haematol.12020 PMid:18322256
- Bessler
M, Mason P, Link D, et al. Inherited bone marrow failure syndromes. In:
DGN, SHO, DG, et al., editors. Nathans and Oski's Hematology of infancy
and childhood. 8. Philadelphia: W. B. Saunders Co; 2014. https://www.ncbi.nlm.nih.gov/pmc/articles/PMC3875142/
- Risitano
AM, Maciejewski JP, Green S, et al. In-vivo dominant immune responses
in aplastic anaemia: molecular tracking of putatively pathogenetic
T-cell clones by TCR beta-CDR3 sequencing. Lancet. 2004;364:355-364. https://doi.org/10.1016/S0140-6736(04)16724-X PMid:15276395
- Kagan
WA, Ascensao JA, Pahwa RN, et al. Aplastic anemia: presence in human
bone marrow of cells that suppress myelopoiesis. Proc Natl Acad Sci U S
A. 1976;73:2890-2894. https://doi.org/10.1073/pnas.73.8.2890 PMid:1085449 PMCid:PMC430791
- Kordasti
S, Marsh J, Al-Khan S, et al. Functional characterization of CD4+ T
cells in aplastic anemia. Blood. 2012;119:2033-2043. https://doi.org/10.1182/blood-2011-08-368308 PMid:22138514
- King
KY, Goodell MA. Inflammatory modulation of HSCs: viewing the HSC as a
foundation for the immune response. Nat Rev Immunol (2011) 11:685-92. https://doi.org/10.1038/nri3062 PMid:21904387 PMCid:PMC4154310
- Brown KE, Young NS. Parvoviruses and bone marrow failure. Stem Cells (1996) 14:151-63. https://doi.org/10.1002/stem.140151 PMid:8991535
- Mishra
B, Malhotra P, Ratho RK, Singh MP, Varma S, Varma N. Human parvovirus
B19 in patients with aplastic anemia. Am J Hematol (2005) 79:166-7. https://doi.org/10.1002/ajh.20347 PMid:15929106
- Morinet
F, Leruez-Ville M, Pillet S, Fichelson S. Concise review: anemia caused
by viruses. Stem Cells (2011) 29:1656-60. https://doi.org/10.1002/stem.725 PMid:21898692
- Young
NS, Calado RT, Scheinberg P. Current concepts in the pathophysiology
and treatment of aplastic anemia. Blood (2006) 108:2509-1 https://doi.org/10.1182/blood-2006-03-010777 PMid:16778145 PMCid:PMC1895575
- Mirantes
C, Passegué E, Pietras EM. Pro-inflammatory cytokines: emerging players
regulating HSC function in normal and diseased hematopoiesis. Exp Cell
Res (2014) 329:248-54 https://doi.org/10.1016/j.yexcr.2014.08.017 PMid:25149680 PMCid:PMC4250307
- de
Bruin AM, Voermans C, Nolte MA. Impact of interferon-γ on
hematopoiesis. Blood (2015) 124:2479-87. https://doi.org/10.1182/blood-2014-04-568451 PMid:25185711
- Helge
D. Hartung, Timothy S. Olson, and Monica Bessler, Acquired Aplastic
Anemia in Children Pediatr Clin North Am. 2013 Dec; 60(6): 1311-1336. https://doi.org/10.1016/j.pcl.2013.08.011 PMid:24237973 PMCid:PMC3894991
- Harrison
A.G., Lin T., Wang P. Mechanisms of SARS-CoV-2 transmission and
pathogenesis. Trends Immunol. 2020;41(12):1100-1115. https://doi.org/10.1016/j.it.2020.10.004 PMid:33132005 PMCid:PMC7556779
- D'Errico
S., Zanon M., Montanaro M., Radaelli D., Sessa F., Di Mizio G., Montana
A., Corrao S., Salerno M., Pomara C. More than pneumonia: distinctive
features of SARS-cov-2 infection. From autopsy findings to clinical
implications: a systematic review. Microorganisms. 2020;8(11) https://doi.org/10.3390/microorganisms8111642 PMid:33114061 PMCid:PMC7690727
- Oran
D.P., Topol E.J. Prevalence of asymptomatic SARS-CoV-2 infection: a
narrative review. Ann. Intern. Med. 2020;173(5):362-367. https://doi.org/10.7326/M20-3012 PMid:32491919 PMCid:PMC7281624
- Kuba
K, Imai Y, Rao S, Gao H, Guo F, Guan B et al (2005) A crucial role of
angiotensin converting enzyme 2 (ACE2) in SARS coronavirus-induced lung
injury. Nat Med 11(8):875-879. https://doi.org/10.1038/nm1267 PMid:16007097 PMCid:PMC7095783
- Li
MY, Li L, Zhang Y, Wang XS (2020) Expression of the SARS-CoV-2 cell
receptor gene ACE2 in a wide variety of human tissues. Infect Dis
Poverty 9(1):45. https://doi.org/10.1186/s40249-020-00662-x PMid:32345362 PMCid:PMC7186534
- Shokrollah
Elahi. Hematopoietic responses to SARS-CoV-2 infection, Cell Mol Life
Sci. 202213;79(3):187. https://doi.org/10.1007/s00018-022-04220-6 PMid:35284964 PMCid:PMC8918078
- Attia
H., El Nagdy M., Abdel HalimR.M.Preliminary study of sCD14 and sCD163
as predictors of disease severity and ICU admission in COVID-19:
Relation to hematological parameters, blood morphological changes and
inflammatory biomarkers. Mediterr J Hematol Infect Dis 2023, 15(1):
e2023046 https://doi.org/10.4084/MJHID.2023.046 PMid:37705527 PMCid:PMC10497305
- Zhi
ZEKZ Subspecialty Group of Hematology, Society of Pediatrics, Chinese
Medical Association the editorial board, Chinese Journal. of
Pediatrics. Recommendations for diagnosis and treatment of acquired
aplastic anemia in children. Chin J Pediatr. 52(2):103-6(2014). https://www.ncbi.nlm.nih.gov/pmc/articles/PMC4280184/
- Camitta
B, Rozman C, Marin P, Nomdedeu B, Montserrat E. Criteria for severe
aplastic anaemia. Lancet.331:303-4 (1988). https://doi.org/10.1016/S0140-6736(88)90388-1 PMid:2893118
- Lesmana
H, Jacobs T, Boals M, Gray N et al.(2021)Eltrombopag in children with
severe aplastic anemia Pediatr Blood Cancer. 68(8):e29066. https://doi.org/10.1002/pbc.29066 PMid:33855784
- Wire
MB, Li X, Zhang J, Sallas W, et al. (2018), Ouatas T Modeling and
simulation support eltrombopag dosing in pediatric patients with immune
thrombocytopenia. Clin Pharmacol Ther 104(6):1199-207. https://doi.org/10.1002/cpt.1066 PMid:29536526
- Angelica
Barone, Annunziata Lucarelli, Daniela Onofrillo et al. (2015),
Marrow Failure Study Group of the Pediatric Haemato-Oncology Italian
Association; Diagnosis and management of acquired aplastic anemia in
childhood. Guidelines from the Marrow Failure Study Group of the
Pediatric Haemato-Oncology Italian Association (AIEOP) Blood Cells Mol
Dis, 55(1):40-7 https://doi.org/10.1016/j.bcmd.2015.03.007 PMid:25976466
- Guan
WJ, Ni ZY, Hu Y, Liang WH, Ou CQ, He JX et al (2020) Clinical
characteristics of coronavirus disease 2019 in China J Emerg Med. 2020
Apr; 58(4): 711-712. https://doi.org/10.1016/j.jemermed.2020.04.004 PMCid:PMC7266766
- Upchurch
DM, Wong MS, Yuan AH, Haderlein TP, McClendon J, Christy A, Washington
DL.COVID-19 infection in the Veterans Health Administration:
gender-specific racial and ethnic differences. Women's Health Issues.
2022;32:41-50. https://doi.org/10.1016/j.whi.2021.09.006 PMid:34702652 PMCid:PMC8486675
- Lee,
N. C. J., Patel, B., Etra, A., Bat, T., Ibrahim, I. F., Vusirikala, M.,
Chen, M., Rosado, F., Jaso, J. M., Young, N. S., & Chen, W. (2022).
SARS-CoV-2 infection associated with aplastic anemia and pure red cell
aplasia. Blood advances, 6(13), 3840-3843. https://doi.org/10.1182/bloodadvances.2022007174 PMid:35452511 PMCid:PMC9040401
- Avenoso
D, Marsh JCW, Potter V, et al. SARS-CoV-2 infection in aplastic
anaemia. Haematologica. 2022;107(2):541-543. https://doi.org/10.3324/haematol.2021.279928 PMid:34670361 PMCid:PMC8804560
- Hock
H, Kelly HR, Meyerowitz EA, Frigault MJ, Massoth LR. Case 31-2021: a
21-year-old man with sore throat, epistaxis, and oropharyngeal
petechiae. N Engl J Med. 2021;385(16):1511-1520. https://doi.org/10.1056/NEJMcpc2027096 PMid:34644476 PMCid:PMC8531984
- Chakravarthy
R, Murphy ML, Ann Thompson M, McDaniel HL, Zarnegar-Lumley S,
Borinstein SC. SARS-CoV-2 infection coincident with newly diagnosed
severe aplastic anemia: a report of two cases. Pediatr Blood Cancer.
2022;69(4):e29433. https://doi.org/10.1002/pbc.29433 PMid:34767303 PMCid:PMC8662128
- Pascutti
MF, Erkelens MN, Nolte MA. Impact of viral infections on hematopoiesis:
from beneficial to detrimental effects on bone marrow output. Front
Immunol. 2016;7:364. https://doi.org/10.3389/fimmu.2016.00364 PMid:27695457 PMCid:PMC5025449
- Terpos
E, Ntanasis-Stathopoulos I, Elalamy I, Kastritis E, Sergentanis TN,
Politou M, Psaltopoulou T, Gerotziafas G, Dimopoulos MA. Hematological
findings and complications of COVID-19. Am J Hematol.
2020;95(7):834-47. https://doi.org/10.1002/ajh.25829 PMid:32282949 PMCid:PMC7262337
- Wang
F, Hou H, Luo Y, Tang G, Wu S, Huang M, Liu W, Zhu Y, Lin Q, Mao L. The
laboratory tests and host immunity of COVID-19 patients with different
severity of illness. JCI insight. 2020; 5(10). https://doi.org/10.1172/jci.insight.137799 PMid:32324595 PMCid:PMC7259533
- Ranjima
M, Gobbur RH. Severe Aplastic Anemia Secondary to SARS CoV-2 Infection-
A Case Report. Journal of Pediatrics, Perinatology and Child Health 5
(2021): 230-237. https://www.fortunejournals.com/articles/severe-aplastic-anemia-secondary-to-sars-cov2-infection-a-case-report.html
- Fatemeh
Nejatifar, Ezat Hesni, Ali Akbar Samadani A Novel Case Report of Severe
Aplastic Anemia with COVID Infection. Ethiop J Health Sci. 2023 Jan;
33(1): 177-181. https://www.ncbi.nlm.nih.gov/pmc/articles/PMC9987292/
- Weiskopf
D, Schmitz KS, Raadsen MP, Grifoni A, Okba NM, Endeman H, van den Akker
JP, Molenkamp R, Koopmans MP, van Gorp EC. Phenotype and kinetics of
SARS-CoV-2-specific T cells in COVID-19 patients with acute respiratory
distress syndrome. Sci Immunol. 2020;5(48):eabd2071.
https://doi.org/10.1126/sciimmunol.abd2071 PMid:32591408 PMCid:PMC7319493
- Wang
F, Hou H, Yao Y, Wu S, Huang M, Ran X, Zhou H, Liu Z, Sun
Z.Systemically comparing host immunity between survived and deceased
COVID-19 patients. Cell Mol Immunol. 2020;17(8):875-7. https://doi.org/10.1038/s41423-020-0483-y PMid:32541836 PMCid:PMC7295144
- Sharma
C, Ganigara M, Galeotti C, Burns J, Berganza FM, Hayes DA, Singh-Grewal
D, Bharath S, Sajjan S, Bayry J. Multisystem inflammatory syndrome in
children and Kawasaki disease: a critical comparison. Nat Rev Rheumatol.
2021;17(12):731-48 https://doi.org/10.1038/s41584-021-00709-9 PMid:34716418 PMCid:PMC8554518
- Martinez
OM, Bridges ND, Goldmuntz E, Pascual V. The immune roadmap for
understanding multisystem inflammatory syndrome in children:
opportunities and challenges. Nat Med. 2020;26(12):1819-24. https://doi.org/10.1038/s41591-020-1140-9 PMid:33139949
- Fara
A, Mitrev Z, Rosalia RA, et al. Cytokine storm and COVID-19: a
chronicle of pro-inflammatory cytokines. Open Biol 10 (2020): 200160.
https://doi.org/10.1098/rsob.200160 PMid:32961074 PMCid:PMC7536084
- Velier
M, Priet S, Appay R, et al. Severe and Irreversible Pancytopenia
Associated With SARS-CoV-2 Bone Marrow Infection in a Patient With
Waldenstrom Macro-globulinemia. Clinical Lymphoma Myeloma and Leukemia
21 (2021): e503-e505 https://doi.org/10.1016/j.clml.2021.01.005 PMid:33563581 PMCid:PMC7832621
- Hernandez
JM, Quarles R, Lakshmi S, et al. Pancytopenia and Profound Neutropenia
as a Sequela of Severe SARS-CoV-2 Infection (COVID-19) With Concern for
Bone Marrow Involvement. Open Forum Infectious Diseases 8 (2021). https://doi.org/10.1093/ofid/ofab017 PMid:33604404 PMCid:PMC7880265
- Smith
JN, Kanwar VS, MacNamara KC. Hematopoietic stem cell regulation by Type
I and II interferons in the pathogenesis of acquired aplastic anemia.
Front Immunol. 2016;7:330. https://doi.org/10.3389/fimmu.2016.00330