Yuxi Ding1,*,
Kun Yang1,*, Xiaodong Liu1,
Jian Xiao1, Wanting Li1
and Huixiu Zhong2.
1 Department of Hematology, Zigong First People's Hospital, Zigong, China.
2 Department of Laboratory Medicine, Zigong First People's Hospital, Zigong, China.
* This author equally contributed to this work.
Correspondence to: Kun
Yang, Department of Hematology, Zigong First People's Hospital, Zigong,
China; ORCID: 0000-0002-8619-9676, E-mail:
1759874951@qq.com
Published: November 01, 2023
Received: September 06, 2023
Accepted: October 16, 2023
Mediterr J Hematol Infect Dis 2023, 15(1): e2023062 DOI
10.4084/MJHID.2023.062
This is an Open Access article distributed
under the terms of the Creative Commons Attribution License
(https://creativecommons.org/licenses/by-nc/4.0),
which permits unrestricted use, distribution, and reproduction in any
medium, provided the original work is properly cited.
|
To the editor
Congenital
sideroblastic anemias (CSAs) are inherited diseases of mitochondrial
dysfunction due to defects in heme biosynthesis, iron-sulfur cluster
biogenesis, generalized mitochondrial protein synthesis, or the
synthesis of specific mitochondrial proteins involved in oxidative
phosphorylation.[1] CSAs are
characterized by the
accumulation of ring sideroblasts in the bone marrow, with ineffective
erythropoiesis and increased serum and tissue iron levels. X-linked
sideroblastic anemia (XLSA) is the most common form of CSA and is
attributed to 5-aminolevulinate synthase (ALAS2) mutations.[2] Here, we presented a de novo mutation in
ALAS2 in a
Chinese patient with CSA and a good response to pyridoxine treatment.
The
proband was a 32-year-old female who was the family's only child. The
family history was unremarkable, and the parents were
non-consanguineous. At age 27, the proband required blood transfusions
during her first pregnancy due to severe anemia. At that time, due to
the low level of vitamin B12, the proband was incorrectly diagnosed
with megaloblastic anemia.
At age 32, the proband again
developed severe anemia during her second pregnancy and required a
blood transfusion. Physical examination revealed that the spleen of the
proband was enlarged to 5 cm below the costal margin. A hemogram at
presentation to our center revealed a hemoglobin level of 5.9 g/dL
(reference value: 12.0–15.0 g/dL), a red blood cell count of 1.41×1012/L
(reference value: 3.8–5.1×1012/L),
a mean corpuscular volume of 127.7 fL (reference value: 80–100 fL),
mean corpuscular hemoglobin of 41.8 pg (reference value: 27–34 pg), a
mean corpuscular hemoglobin concentration of 32.8 g/dL (reference
value: 31.6–35.4 g/dL), a reticulocyte percentage of 2.35% (reference
value: 0.5–1.5%), a white blood cell count of 3.95×109/L (reference
value: 3.5–9.5×109/L),
and a platelet count of 132×109/L (reference
value: 100–300×109/L). A
peripheral blood smear was dimorphic, including microcytic hypochromic,
normocytic, and normochromic erythrocytes (Figure 1A).
Serum biochemical tests revealed a total bilirubin level of 15 μmol/L
(reference value: 0–23 μmol/L), an indirect bilirubin level of 8.7
μmol/L (normal range: 5–18 μmol/L), and a lactate dehydrogenase level
of 117 U/L (reference value: 120–250 U/L). Iron metabolism testing
revealed that serum ferritin, serum iron, and transferrin were 751.4
ng/mL (reference value: 4.63–204 ng/mL), 37.18 μmol/L (reference value:
7–30 μmol/L), and 1.57 g/L (reference value: 1.7–3.4 g/L),
respectively. Levitt’s CO breath test showed that the erythrocyte life
span of the proband was 24 days (normal range: 70–140 days). Laboratory
tests for megaloblastic anemia, autoimmune hemolytic anemia, paroxysmal
nocturnal hemoglobinuria, glucose-6-phosphate dehydrogenase deficiency,
thalassemia, and hepatopathy were negative.
Next-generation
sequencing of the proband and her family was carried out to investigate
underlying variants associated with anemia in the proband. A de novo
heterozygous missense mutation of ALAS2:
c.1439G>A was found and further confirmed by Sanger sequencing (Figure 1B).
The c.1439G>A mutation led to a substitution of a conserved
arginine
to histidine at residue 480 (p.Arg480His) in exon 9 of the ALAS2
protein. Given the ALAS2
mutation, a diagnosis of CSA was suspected.
Bone marrow analysis revealed marked erythroid hyperplasia with
increased pronormoblast and basophilic erythroblast (Figure 1C).
In Prussian blue-stained specimens, a bone marrow smear showed
erythroid hyperplasia with a 66% proportion of total sideroblasts, and
the proportion of ring sideroblasts was 16% (Figure 1D).
Chromosome analysis revealed a normal karyotype (46, XY). A bone marrow
biopsy showed hyperactive hyperplasia and no increase in reticulin
fibers (MF0). Following the diagnosis of SA in the bone marrow, further
investigations were conducted, including tests for pyridoxine, zinc,
lead, and copper levels and chromosomal microarray analysis for
myelodysplastic syndromes. All tests came back normal. The proband was
thus diagnosed with CSA caused by this mutation of the ALAS2 gene. The
proband had a good response to pyridoxine treatment, but her ferritin
level gradually rose to 925.85 ng/mL.
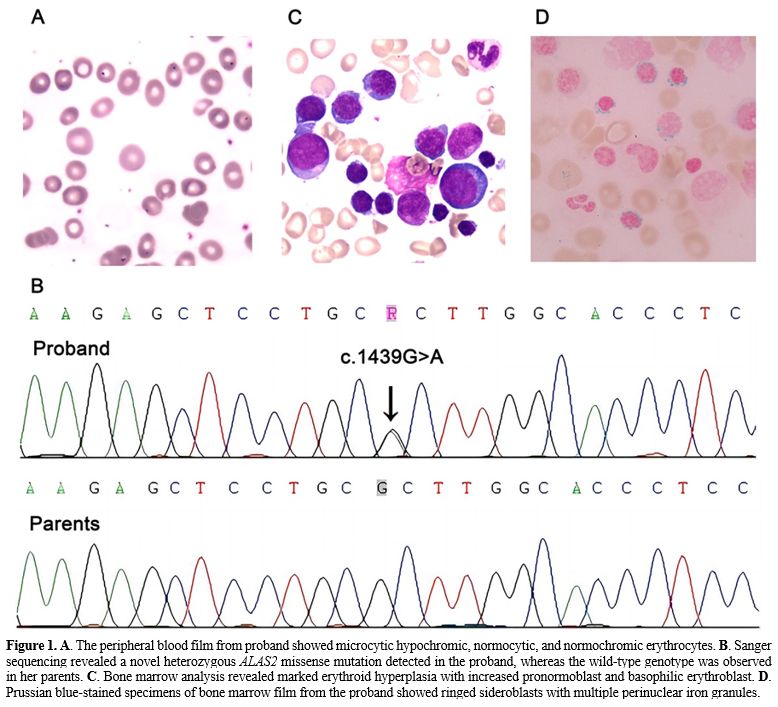 |
- Figure
1. A. The
peripheral blood film from proband showed microcytic hypochromic,
normocytic, and normochromic erythrocytes. B.
Sanger sequencing
- revealed a novel heterozygous ALAS2 missense mutation
detected in the proband, whereas the wild-type genotype was observed in
her parents. C. Bone
- marrow analysis revealed marked erythroid hyperplasia with increased
pronormoblast and basophilic erythroblast. D.
Prussian blue-stained specimens
- of bone marrow film from the proband
showed ringed sideroblasts with multiple perinuclear iron
granules.
|
XLSA
typically affects younger males due to an X-linked recessive pattern of
inheritance. However, as a result of familial-skewed inactivation of
the normal X chromosome, females with ALAS2 mutations may
have a late-onset clinical phenotype, as with the proband in this
study.[3]
The management of CSA remains primarily supportive rather than
definitive. ALAS2 catalyzes the first step of the heme biosynthetic
pathway by condensing glycine and succinyl-CoA to form
delta-aminolevulinic acid in the presence of pyridoxal 5’-phosphate,
which is the metabolite of vitamin B6.[4]
Pyridoxine
can enhance the activity of the ALAS2 enzyme, and more than half of
XLSA patients are responsive to supplementation with pyridoxine.[5] More than 100 distinct mutations in
the ALAS2
gene have been reported. Most disease-associated variants occur in
exons 5 and 9; the latter contains the pyridoxal-binding amino acid.[2] This finding was confirmed by the
good response to pyridoxine treatment observed in the proband.
Patients
with CSA are prone to iron overload, whether pyridoxine-responsive or
not, regardless of red blood cell transfusions. Iron overload is partly
attributed to reduced hepcidin level secondary to ineffective
erythropoiesis, which promotes intestinal iron absorption.[6]
The ferritin level of our reported XLSA patient increased gradually. It
is therefore necessary to monitor regularly patients' clinical,
laboratory, and radiological parameters to detect these long-term
complications. Anecdotal reports of hematopoietic stem cell
transplantation in CSA describe effective remission.[7]
However, early diagnosis and management of CSA remain fundamental,
especially as iron overload should be kept at a minimum to ensure a
better outcome of a potential future transplantation. Additionally,
developing definitive treatments for CSA is an area of need.
Preclinical studies and clinical trials are essential to determine
whether novel agents such as luspatercept or approaches involving gene
therapy for CSA would benefit its treatment.[8]
In conclusion, we identified a novel ALAS2
missense mutation causing CSA in the Chinese population. Our findings
will provide valuable insights to broaden the clinical phenotypic
spectrum of CSA and improve understanding of ALAS2 gene variants.
Acknowledgments
We thank the
proband and her family members for their continuous support and
participation in this study.
Funding
This
study was financially supported by the Key Science and Technology
Project of Zigong (grant nos. 2020YXY04).
Ethics approval
The study protocol was approved by the
Medical Ethics Committee of the First People’s Hospital of Zigong.
Informed
consent
Written informed consent was
obtained from the participating family.
References
- Fleming MD. Congenital
sideroblastic anemias: iron
and heme lost in mitochondrial translation. Hematology Am Soc Hematol
Educ Program. 2011;2011:525-31. https://doi.org/10.1182/asheducation-2011.1.525
PMid:22160084
- Ducamp
S, Fleming MD. The molecular genetics of sideroblastic anemia. Blood.
2019;133:59-69. https://doi.org/10.1182/blood-2018-08-815951
PMid:30401706 PMCid:PMC6318428
- Cazzola
M, May A, Bergamaschi G, Cerani P, Rosti V, Bishop DF. Familial-skewed
X-chromosome inactivation as a predisposing factor for late-onset
X-linked sideroblastic anemia in carrier females. Blood.
2000;96:4363-5. https://doi.org/10.1182/blood.V96.13.4363
PMid:11110715
- Shoolingin-Jordan
PM, Al-Daihan S, Alexeev D, Baxter RL, Bottomley SS, Kahari ID, Roy I,
Sarwar M, Sawyer L, Wang SF. 5-Aminolevulinic acid synthase: mechanism,
mutations and medicine. Biochim Biophys Acta. 2003;1647:361-6. https://doi.org/10.1016/S1570-9639(03)00095-5
PMid:12686158
- Bottomley
SS, Fleming MD. Sideroblastic anemia: diagnosis and management. Hematol
Oncol Clin North Am. 2014;28:653-70, v. https://doi.org/10.1016/j.hoc.2014.04.008
PMid:25064706
- Furuyama
K, Kaneko K. Iron metabolism in erythroid cells and patients with
congenital sideroblastic anemia. Int J Hematol. 2018;107:44-54. https://doi.org/10.1007/s12185-017-2368-0
PMid:29139060
- Kim
MH, Shah S, Bottomley SS, Shah NC. Reduced-toxicity allogeneic
hematopoietic stem cell transplantation in congenital sideroblastic
anemia. Clin Case Rep. 2018;6:1841-4. https://doi.org/10.1002/ccr3.1667
PMid:30214775 PMCid:PMC6132150
- Van
Dijck R, Goncalves Silva AM, Rijneveld AW. Luspatercept as Potential
Treatment for Congenital Sideroblastic Anemia. N Engl J Med.
2023;388:1435-6. https://doi.org/10.1056/NEJMc2216213
PMid:37043658