Sonia Alexiadou1, Christina Tsigalou2, Eleni Kourkouni3, Aggelos Tsalkidis1 and Elpis Mantadakis1.
1
Department of Pediatrics, Democritus University of Thrace, University General Hospital of Alexandroupolis, Thrace, Greece
2 Laboratory of Hygiene and Environmental Protection, Democritus University of Thrace, Alexandroupolis, Thrace, Greece
3 Centre for Clinical Epidemiology and Outcomes Research (CLEO), Athens, Greece
Correspondence to:
Elpis Mantadakis, MD, PhD. Professor of Pediatrics-Pediatric
Hematology/ Oncology. Democritus University of Thrace Faculty of
Medicine. Head, Department of Pediatrics. University General Hospital
of Alexandroupolis. Alexandroupolis, Thrace, Greece.Tel: +30-25513-51424, E-mail:
emantada@med.duth.gr
Published: November 01, 2024
Received: August 14, 2024
Accepted: October 10, 2024
Mediterr J Hematol Infect Dis 2024, 16(1): e2024075 DOI
10.4084/MJHID.2024.075
This is an Open Access article distributed
under the terms of the Creative Commons Attribution License
(https://creativecommons.org/licenses/by-nc/4.0),
which permits unrestricted use, distribution, and reproduction in any
medium, provided the original work is properly cited.
|
Abstract
Background:
Iron deficiency anemia (IDA) is a major public health problem among
children worldwide. Iron deficiency without anemia (IDWA) is at least
twice as common as IDA. Some studies propose that oral iron
fortification can modify the infant's gut microbiome, leading to
intestinal inflammation. Objectives: To determine whether oral iron therapy can lead to intestinal inflammation in children with IDA or IDWA. Patients and methods:
Fifty-six patients aged 6 months to 16 years (median age 7.6 years)
with IDA or IDWA were randomly assigned to receive either iron
(III)-hydroxide polymaltose complex (IPC) 5 mg/kg once daily (maximum
dose 100 mg) or sucrosomial iron (SI)1.4 mg/kg once daily (maximum dose
29.4 mg). Safety and efficacy were studied after 30 and 90 days of
treatment. In addition, fecal calprotectin as a marker of intestinal
inflammation was measured simultaneously and compared to results
obtained before therapy. Results:
A significant increase in serum ferritin was noted in both groups as
the median ferritin level at baseline was 6.7 μg/L in the IPC group and
6.6 μg/L in the SI group, increasing to 15.9 μg/L and 12.1 μg/L
respectively, after 90 days of treatment. However, there was no
significant change in fecal calprotectin in either group. In addition,
no differences in the trend over time were observed between the two
groups regarding fecal calprotectin, serum ferritin, and hemoglobin. Conclusions:
IPC and SI were equally effective in treating IDA and IDWA. At the
recommended doses, oral iron therapy does not seem to induce intestinal
inflammation.
|
Introduction
One-fourth of the global population has been estimated to be anemic, as in 2021, 1.92 billion people globally had anemia.[1]
Across all ages and both sexes, the leading cause of anemia is dietary
iron deficiency.[1] Therefore, iron deficiency anemia (IDA) is by far the
most common anemia worldwide. For every patient with IDA, there is at
least one more with iron deficiency without anemia (IDWA), and most of
them reside in countries with limited resources.[2] In
developed countries, the prevalence of IDA in children < 4 years of
age is estimated to be 20.1%, while the prevalence increases to 39% in
developing countries.[3]
Iron supplementation
aims to prevent sideropenia and to correct established IDA. Many oral
iron products at various formulations and doses are available,
including ferrous iron salts, ferric iron salts, heme-iron
polypeptides, carbonyl iron, chelates of iron with amino acids,
complexes of ferric iron with polysaccharides (iron (III)-hydroxide
polymaltose complex, IPC), and complexes of iron with amino acids in
casein, such as iron protein succinylate and iron acetyl aspartylate.[2]
Ferrous salts, either sulfate or gluconate, are by far the most
commonly used oral iron preparations. However, absorption of oral iron
is poor and may be further reduced by certain medications (e.g., proton
pump inhibitors) and food intake, thus making oral iron treatment
difficult.[4,5] In addition, gastrointestinal adverse
effects diminish compliance with therapy. A recent study by Jaeggi et
al. showed that the provision of iron-containing micronutrient powders
(MNPs) to weaning infants adversely affects the gut microbiome,
increasing pathogen abundance, fecal calprotectin, and rates of
diarrhea among Kenyan infants.[6]
Recently, new
preparations of oral iron, such as sucrosomial iron (SI) became
commercially available. SI is an innovative oral iron formulation in
which ferric pyrophosphate is protected by a phospholipid bilayer,
mainly from sunflower lecithin, plus a sucrester matrix (sucrosome),
which is absorbed through para-cellular and trans-cellular routes (M
cells).[5] To date, in vitro studies have shown that
SI is mostly absorbed as a vesicle-like structure, bypassing the
conventional iron absorption pathway.[5] Due to its
behavior in the gastrointestinal tract, SI is well tolerated and highly
bioavailable, compared to conventional iron salts.[5,7]
In an Italian randomized trial, Pisani et al. showed that oral
liposomal iron was a safe and efficacious alternative to IV iron
gluconate in correcting anemia in patients with chronic kidney disease
(CKD). However, its effects on the repletion of iron stores and the
stabilization of hemoglobin (Hb) after drug discontinuation were
inferior.[8] Adequate data regarding the efficacy of SI in the treatment of children with IDA are still lacking.
Our
study aimed to determine the efficacy and safety of two oral iron
preparations in pediatric patients with IDA or IDWA, particularly on
intestinal inflammation. One of the products, i.e., IPC has been widely
used and studied in the past, while the other (SI), is a new and
promising product with limited safety and efficacy data in children.
Patients and Methods
According
to the World Health Organization (WHO), in assessing anemia, the
following cutoff values for Hb were used: Hb<11 g/dL in children 6
months to 59 months old, Hb<11.5 g/dL for children 5-11 years old,
Hb< 12 g/dL for children 12-14 years old, Hb< 12 g/dL for
adolescent girls > 15 years old, and Hb <13 g/dL for adolescent
boys> 15 years of age.[9]
Patients with IDA had lower Hb values
than those listed above and serum ferritin ≤15 μg/L and/or total iron
binding capacity (TIBC) ≥425 μg/dL. Patients with normal Hb but serum
ferritin ≤15 μg/L or total iron binding capacity (TIBC) ≥425 μg/dL were
defined as patients with IDWA. Exclusion criteria were a previous
allergic reaction to any of the oral iron products used, children with
IDA due to inflammatory bowel diseases (IBD), celiac disease or CKD,
and children on medications that can lower gastric acidity, such as
proton pump inhibitors. The duration of treatment was three months.
Patients were randomly assigned by using a random number-generating
computer program to receive either IPC 5 mg/kg once daily (maximum
daily dose 100 mg) or SI 1.4 mg/kg once daily (maximum daily dose 29.4
mg).
At baseline and after 30 and 90 days, venous blood was drawn.
At the same time, parents were instructed to collect and bring a stool
sample from their children. The following parameters were determined:
Hb, reticulocytes (Ret), serum ferritin, TIBC, serum iron, transferrin
saturation, C-reactive protein (CRP), and erythrocyte sedimentation
rate (ESR). The stool samples were introduced within a special vial
that can preserve feces for 7 days at 2-8 °C and at -20 ºC for 6
months. Calprotectin Turbilatex®, a latex turbidimetric assay for the
quantitative detection of calprotectin in human stool samples, was used
to determine fecal calprotectin. This assay is performed on the
Architect c8000 chemical analyzer (Abbott, Abbott Park, IL, USA). The
calprotectin range is 20-8000 μg/g of stool, with normal levels
indicating the absence of inflammation to be < 50 μg/g.
The
study was approved by the ethics and research committees of the
University General Hospital of Alexandroupolis, and the parents of all
participating children gave written informed consent.
Statistical
Analysis. The chi-square test was also used to test for significant
differences in dichotomous variables. Nominal variables are presented
as absolute and relative (%) frequencies, while quantitative ones with
mean, standard deviation, median, and interquartile range (IQR). The
normality of data was evaluated graphically with histograms, as well as
with the Shapiro-Wilk statistical test. To identify significant changes
in laboratory measurements 90 days after treatment initiation, a paired
t-test (or sign-rank test, when the normality of data did not hold) was
performed. Independent samples t-test (or Mann-Whitney test, when the
normality of data did not hold) was used to compare changes between the
two groups. Trends over time (baseline measurements, 30 and 90 days
after treatment initiation) and differences in trends between the two
groups were evaluated using Linear Mixed Models. The chi-square test of
independence was used to detect differences in the frequency of side
effects between the two groups. The level of statistical significance
was set at 0.05. Analysis was conducted with the statistical package
STATA SE v.18.
Results
Overall,
56 children were enrolled in the study (age 6 months to 15 years). The
demographic characteristics of the participating children are shown in Table 1.
Three of them (girls 9, 11, and 14 years old) did not complete the
designated oral treatment, as they had severe IDA that did not
adequately respond to oral iron therapy after 30 days, thus
necessitating the administration of parenteral iron. In addition, six
patients (1 20-month-old boy, 1 9-year-old boy, and 4 girls 11, 12, 13,
and 15 years old) failed to provide stool samples at 30 and/or 90 days
and were also excluded. Hence, the final analysis included 47 patients.
Among them, 23 patients were prescribed IPC and 24 SI.
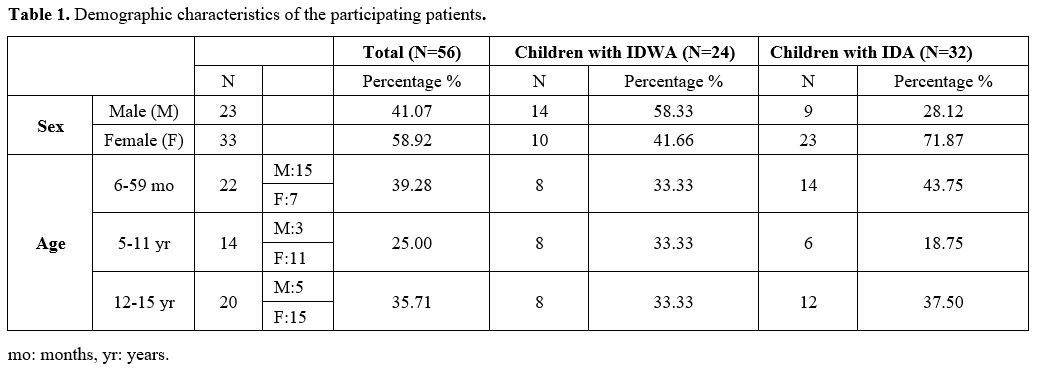 |
- Table 1. Demographic characteristics of the participating patients.
|
In summation,
this case could be used as new evidenceResults for fecal calprotectin, serum ferritin, and Hb at baseline and after 30 and 90 days of treatment are presented in Table 2. Fecal calprotectin levels did not increase compared to baseline measurements in both groups after 90 days of treatment (Figure 1). IPC and SI effectively elevated serum ferritin levels after 30 and 90 days (Figure 2).
Median ferritin level at baseline was 6.7 μg/L in the IPC group and 6.6
μg/L in the SI group, increasing to 15.9 μg/L and 12.1 μg/L
respectively, after 90 days of treatment (p=0.002 and p=0.001, respectively). Both IPC and SI effectively elevated Hb levels in patients with IDA after 30 (p= 0.003 and p<0.001, respectively) and 90 days of treatment (p=0.002 and p=0.003, respectively) (Figure 3).
In addition, no differences in the trend over time were observed
between the two groups regarding fecal calprotectin, serum ferritin,
and Hb (Table 3).
Gastrointestinal
side effects, such as abdominal pain, diarrhea, and constipation, were
reported by 5 patients, 4 in the IPC (17.4%) and 1 (4.2%) in the SI
group (p=0.19).
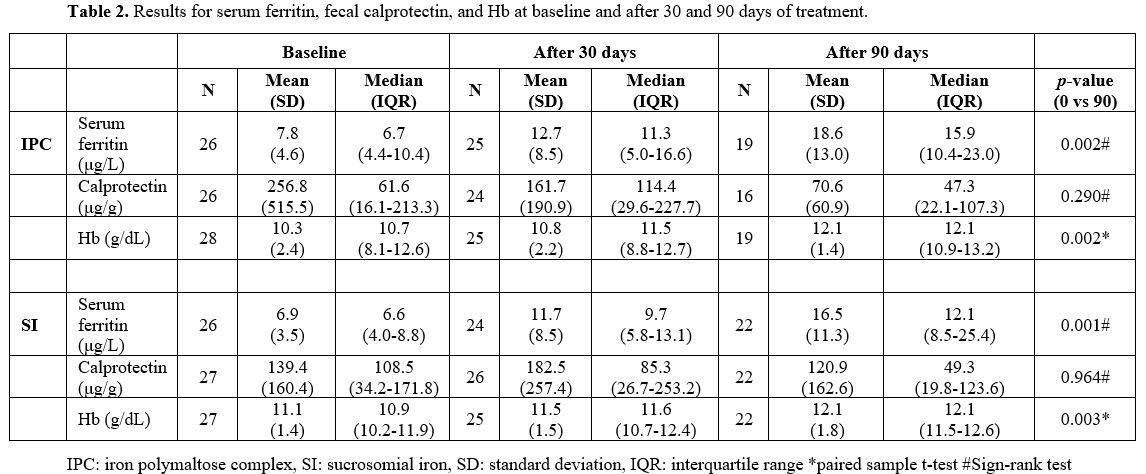 |
Table 2. Results for serum ferritin, fecal calprotectin, and Hb at baseline and after 30 and 90 days of treatment. |
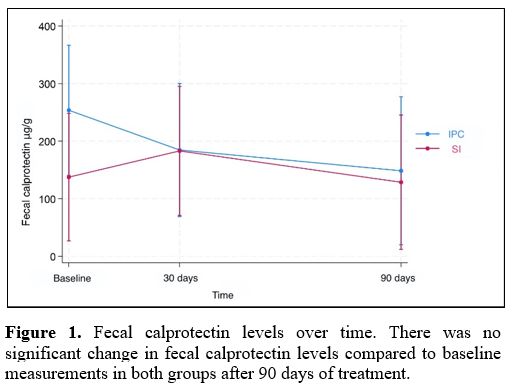 |
Figure 1.
Fecal calprotectin levels over time. There was no significant change in
fecal calprotectin levels compared to baseline measurements in both
groups after 90 days of treatment. |
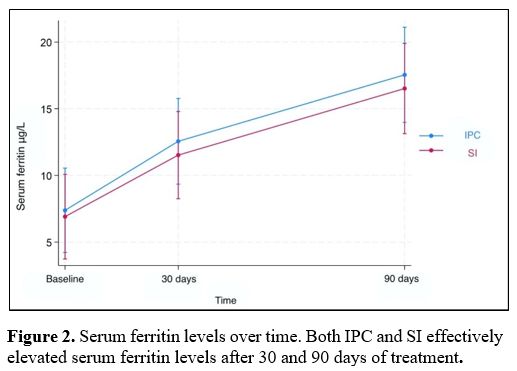 |
Figure 2.
Serum ferritin levels over time. Both IPC and SI effectively elevated
serum ferritin levels after 30 and 90 days of treatment. |
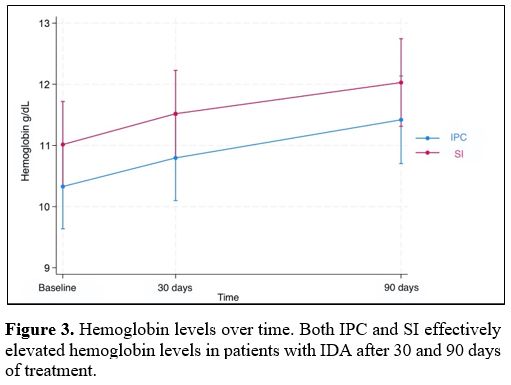 |
Figure 3.
Hemoglobin levels over time. Both IPC and SI effectively elevated
hemoglobin levels in patients with IDA after 30 and 90 days of
treatment. |
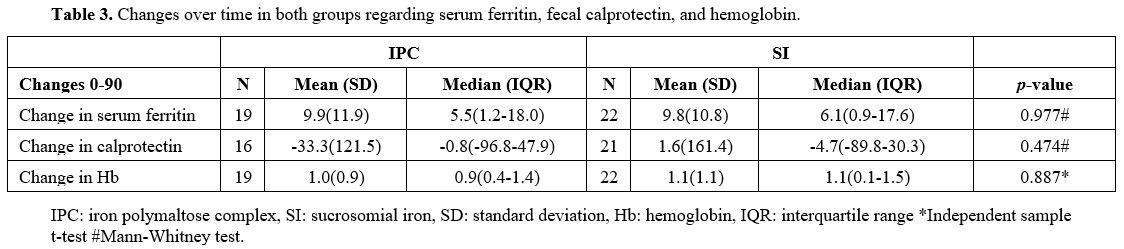 |
Table 3. Changes over time in both groups regarding serum ferritin, fecal calprotectin, and hemoglobin.
|
Discussion
Strategies
to control sideropenia include daily and intermittent iron
supplementation, home fortification with MNPs, fortification of staple
foods and condiments, and activities to improve food security and
dietary diversity.[10] In areas where the prevalence of anemia in children < 5 years of age is ≥20%,
WHO recommends fortification of complementary foods with
iron-containing MNPs in infants and young children aged 6-23 months.[11] In this age group, MNPs decrease the risk of anemia by 18% and iron deficiency by 53% compared to no intervention or placebo.[11]
However, the safety of this strategy is unclear. In a review of 29
studies conducted in low- and middle-income countries in Asia, Africa,
Latin America, and the Caribbean, where anemia is a major public health
problem, MNPs appeared to improve some cognitive outcomes, such as
receptive and expressive language but did not seem to influence
all-cause morbidity, diarrhea, upper respiratory infections or the risk
of malaria.[12,13] However, information on the side
effects and morbidity, including malaria and diarrhea, was scarce, and
therefore, these results should be interpreted with caution.[12]
Management
guidelines and treatment recommendations for iron-deficient children
are not evidence-based, and few clinical studies compare oral iron
products in pediatric patients,[2,14] often making the choice and dose of the product to be used difficult.
The
usually recommended dose for IPC products is 3-6 mg/kg/day in one or
divided doses. Jaber et al. compared the efficacy and safety of iron
gluconate (IG) versus IPC in the prevention of IDA in 105 healthy
infants attending a community pediatric center in Israel. Mean Hb
levels at the study end were significantly higher in the IG group, but
IPC was associated with significantly fewer adverse events.[15]
Name et al. randomized 20 children with IDA in Brazil aged 1 to 13
years to IPC or iron bis-glycinate at 3 mg/kg/day for 45 days. They
reported that both iron preparations were equally effective in
elevating Hb, but only iron bis-glycinate significantly increased
ferritin, suggesting greater efficacy than IPC in increasing iron
stores.[16] Bopche et al. assessed the clinical
response and side effects of ferrous sulfate (FS) and IPC in 106
children with IDA. All patients were given elemental iron 6 mg/kg/day
in three divided doses. Patients who received FS had significantly
higher Hb and fewer residual complaints compared to those who received
IPC. However, gastrointestinal side effects were 2.5 times more common
in the FS-treated children.[17] Powers et al.
randomized 80 infants and children aged 9 to 48 months with nutritional
IDA to 3 mg/kg/day of elemental iron once daily for three months as
either FS or IPC drops. Treatment with FS resulted in a greater
increase in Hb concentration at 12 weeks, while the proportion of
children with complete resolution of IDA at the end of therapy was also
significantly higher in the FS group (29% versus 6%). Both iron
products were well tolerated, but there were significantly more reports
of diarrhea in the IPC group.[18] Sheikh et al.
randomized 70 toddlers with IDA to receive FS or IPC at 6 mg/kg/day of
elemental iron in three divided doses. Response and compliance with
therapy were comparable for both groups.[19] In a
systematic review and meta-analysis that assessed the effectiveness of
IPC in the treatment and prevention of IDA in children, 6 trials (5
from Turkey and 1 from India) were included in the comparison group,
which was used to evaluate IPC versus FS. There was moderate to high
certainty evidence that FS is superior to IPC in correcting IDA, with a
clinically meaningful difference in improving Hb and ferritin levels.
The occurrence of gastrointestinal side effects such as spitting,
vomiting, constipation, nausea, and stomachaches was comparable between
IPC and FS.[20] Russo et al. prospectively monitored
oral iron therapy in children with IDA aged 3 months to 12 years
treated in 15 centers of the Associazione Italiana di Ematologia ed
Oncologia Pediatrica. In this multicenter study, 13 patients with mild
IDA were given liposomal iron. None of them reported gastrointestinal
side effects, such as nausea, vomiting, abdominal pain, or
constipation. However, liposomal iron was less effective in correcting
IDA at 2 and 8 weeks compared to other iron preparations, i.e., ferrous
gluconate, FS, ferric iron salts, and bis-glycinate iron.[21]
Cancelo-Hidalgo et al. performed a systematic review of the
tolerability of different iron supplements and found that ferrous
fumarate had the highest rate of adverse events (47%), followed by FS
and ferrous gluconate (32% and 30.9%, respectively). Among all oral
iron products, ferrous glycine sulfate, iron protein succinylate, and
FS combined with mucoproteose were those better tolerated.[22]
In
our study, patients who were treated with IPC were prescribed 5
mg/kg/day in a single dose. For SI, the dose of 1.4 mg/kg/day was
chosen based on an ex vivo and in vivo study of SI intestinal
absorption and bioavailability[7] and a previously published study.[21]
The doses used for IPC and SI were equally effective in elevating Hb
and serum ferritin levels at 30 and 90 days. The fact that only one
patient in the SI group reported gastrointestinal side effects versus
five in the IPC group was not statistically significant, but in larger
studies, this may not be the case.
Our study showed that oral iron
therapy in children with IDA or IDWA aged 6 months to 16 years (median
age 7.6 years) does not induce intestinal inflammation, as assessed by
fecal calprotectin. Some studies have shown that iron fortification can
extensively modify the gut microbiota, increasing enterobacteria and
decreasing lactobacilli and leading to intestinal inflammation measured
by fecal calprotectin.[6,23] Jaeggi
et al. performed two double-blinded randomized clinical trials in
6-month-old Kenyan infants consuming home-fortified maize porridge
daily for four months. In the first trial, infants received an MNP
containing 2.5 mg of iron as NaFeEDTA or the same MNP without iron. In
the second trial, they received a different MNP containing 12.5 mg of
elemental iron as ferrous fumarate or the same MNP without iron.
Comparing +FeMNP versus −FeMNP by qPCR analysis, they revealed a
borderline significant effect of iron on enterobacteria, with higher
concentrations in the +FeMNP group versus the −FeMNP group. There was a
significant treatment effect on the sum of the pathogenic E. coli
at the endpoint, with higher concentrations in those who received
iron-fortified MNPs. They concluded that the provision of
iron-containing MNPs to weaning infants adversely affects the gut
microbiome, increasing pathogen abundance, and they also reported
increased fecal calprotectin, as well as increased rates of diarrhea
among the +FeMNPs groups.6 However, there were no significant
correlations of fecal calprotectin with the overall gut microbiome
composition assessed by pyrosequencing. The results of our study, at
first glance, may seem conflicting. However, the increase in fecal
calprotectin through iron fortification shown in the Kenyan study was
significant in infants who were iron-sufficient at baseline, not in
infants with iron deficiency, like the participants in our study.
A
wide range in fecal calprotectin concentration was noted among our
patients, decreasing the power of the study. Calprotectin is derived
predominantly from neutrophils, where it accounts for about 60% of the
cytosolic protein, with a lesser contribution from monocytes and
macrophages.[24] Fecal calprotectin correlates with
the number of neutrophils present in the intestinal lumen and thus
allows the detection of an acute gastrointestinal inflammatory
response. Moreover, it can be retrieved non-invasively, is inexpensive,
and remains stable at room temperature in the stools for at least three
days.[25] However, concentrations in the feces of healthy infants are much higher than those observed in children older than 4 years.[26]
Moreover, there are a lot of potential factors that can modify fecal
calprotectin, such as viral or bacterial gastrointestinal infections,
which are relatively common in children, or the use of drugs such as
NSAIDs.[25,27] Considering the above, the wide range of fecal calprotectin levels observed in our study may not be surprising.
Our
study has some limitations. First, the doses of the two oral iron
preparations used may not have been equivalent, although we used the
best published and available evidence for choosing equivalent doses.
Second, the sample size of our study was relatively small, possibly
affecting some outcomes, such as the frequency of gastrointestinal side
effects. To this extent, larger studies are needed to conclusively
assess the safety profile of SI in comparison to IPC and other oral
iron products. Finally, the collection of data on gastrointestinal side
effects was based on parental reports alone, which may have been biased.
Conclusions
IDA
remains a major public health problem with high prevalence in children.
Oral iron therapy in children with IDWA or IDA does not seem to induce
intestinal inflammation, as assessed by fecal calprotectin, and
therefore, it should be used without delay. Several oral iron products
are available, but formulation, dosage, and duration of treatment are
mainly empirical. SI is a relatively new oral iron preparation that
appears to be a safe and effective alternative to IPC. Large and
well-designed clinical trials are needed to establish specific
evidence-based guidelines for the treatment of IDWA and IDA in
pediatric patients
References
- GBD 2021 Anaemia Collaborators. Prevalence, years
lived with disability, and trends in anaemia burden by severity and
cause, 1990-2021: findings from the Global Burden of Disease Study
2021. Lancet Haematol. 2023;10:e713-e734. https://doi.org/10.1016/S2352-3026(23)00160-6
- Mantadakis
E, Chatzimichael E, Zikidou P. Iron Deficiency Anemia in Children
Residing in High and Low-Income Countries: Risk Factors, Prevention,
Diagnosis and Therapy. Mediterr J Hematol Infect Dis.
2020;12(1):e2020041. https://doi.org/10.4084/mjhid.2020.041
- Martinez-Torres
V, Torres N, Davis JA, Corrales-Medina FF. Anemia and Associated Risk
Factors in Pediatric Patients. Pediatric Health Med Ther.
2023;14:267-280. https://doi.org/10.2147/PHMT.S389105
- Muñoz
M, Gómez-Ramírez S, Bhandari S. The safety of available treatment
options for iron-deficiency anemia. Expert Opin Drug Saf.
2018;17(2):149-159. https://doi.org/10.1080/14740338.2018.1400009
- Gómez-Ramírez
S, Brilli E, Tarantino G, Muñoz M. Sucrosomial® Iron: A New Generation
Iron for Improving Oral Supplementation. Pharmaceuticals (Basel).
2018;11(4):97. https://doi.org/10.3390/ph11040097
- Jaeggi
T, Kortman GA, Moretti D, Chassard C, Holding P, Dostal A, Boekhorst J,
Timmerman HM, Swinkels DW, Tjalsma H, Njenga J, Mwangi A, Kvalsvig J,
Lacroix C, Zimmermann MB. Iron fortification adversely affects the gut
microbiome, increases pathogen abundance, and induces intestinal
inflammation in Kenyan infants. Gut. 2015;64(5):731-42. https://doi.org/10.1136/gutjnl-2014-307720
- Fabiano
A, Brilli E, Fogli S, Beconcini D, Carpi S, Tarantino G, Zambito Y.
Sucrosomial® iron absorption studied by in vitro and ex-vivo models.
Eur J Pharm Sci. 2018;111:425-431. https://doi.org/10.1016/j.ejps.2017.10.021
- Pisani
A, Riccio E, Sabbatini M, Andreucci M, Del Rio A, Visciano B. Effect of
oral liposomal iron versus intravenous iron for treatment of iron
deficiency anaemia in CKD patients: a randomized trial. Nephrol Dial
Transplant. 2015;30(4):645-52. https://doi.org/10.1093/ndt/gfu357
- Guideline
on haemoglobin cutoffs to define anaemia in individuals and populations
[Internet]. Geneva: World Health Organization; 2024. PMID: 38530913
- Pasricha
SR, Drakesmith H, Black J, Hipgrave D, Biggs BA. Control of iron
deficiency anemia in low- and middle-income countries. Blood.
2013;121(14):2607-17. https://doi.org/10.1182/blood-2012-09-453522
- WHO
Guideline: Use of Multiple Micronutrient Powders for Point-of-Use
Fortification of Foods Consumed by Infants and Young Children Aged 6-23
Months and Children Aged 2-12 Years. Geneva: World Health Organization;
2016. PMID: 28079999. https://iris.who.int/bitstream/handle/10665/252540/9789241549943-eng.pdf?sequence=1
- De-RegilLM,
Suchdev PS, Vist GE, Walleser S, Peña-Rosas JP. Home fortification of
foods with multiple micronutrient powders for health and nutrition in
children under two years of age (Review). Evid Based Child Health.
2013;8(1):112-201. https://doi.org/10.1002/ebch.1895
- Lopez
de Romaña D, Mildon A, Golan J, Jefferds MED, Rogers LM, Arabi M.
Review of intervention products for use in the prevention and control
of anemia. Ann N Y Acad Sci. 2023;1529(1):42-60. https://doi.org/10.1111/nyas.15062
- Buchanan
GR. Paucity of clinical trials in iron deficiency: lessons learned from
the study of VLBW infants. Pediatrics. 2013;131(2):e582-4. https://doi.org/10.1542/peds.2012-3365
- Jaber
L, Rigler S, Taya A, Tebi F, Baloum M, Yaniv I, Haj Yehia M, Tamary H.
Iron polymaltose versus ferrous gluconate in the prevention of iron
deficiency anemia of infancy. J PediatrHematol Oncol. 2010;32(8):585-8.
https://doi.org/10.1097/MPH.0b013e3181ec0f2c
- Name
JJ, Vasconcelos AR, Valzachi Rocha Maluf MC. Iron Bisglycinate Chelate
and Polymaltose Iron for the Treatment of Iron Deficiency Anemia: A
Pilot Randomized Trial. Curr Pediatr Rev. 2018;14(4):261-268. https://doi.org/10.2174/1573396314666181002170040
- Bopche
AV, Dwivedi R, Mishra R, Patel GS. Ferrous sulfate versus iron
polymaltose complex for treatment of iron deficiency anemia in
children. Indian Pediatr. 2009;46(10):883-5. PMID:19430060. https://www.indianpediatrics.net/oct2009/883.pdf
- Powers
JM, Buchanan GR, Adix L, Zhang S, Gao A, McCavit TL. Effect of Low-Dose
Ferrous Sulfate vs Iron Polysaccharide Complex on Hemoglobin
Concentration in Young Children With Nutritional Iron-Deficiency
Anemia: A Randomized Clinical Trial. JAMA. 2017;317(22):2297-2304. https://doi.org/10.1001/jama.2017.6846
- Sheikh
MA, Shah M, Shakir MU. Comparison of efficacy of ferrous sulfate and
iron polymaltose complex in the treatment of childhood iron deficiency
anemia. PJMHS. 2017;11: 259-261. https://pjmhsonline.com/2017/jan_march/pdf/259.pdf
- Mohd
Rosli RR, Norhayati MN, Ismail SB. Effectiveness of iron polymaltose
complex in treatment and prevention of iron deficiency anemia in
children: a systematic review and meta-analysis. PeerJ. 2021;9:e10527. https://doi.org/10.7717/peerj.10527
- Russo
G, Guardabasso V, Romano F, Corti P, Samperi P, Condorelli A, Sainati
L, Maruzzi M, Facchini E, Fasoli S, Giona F, Caselli D, Pizzato C,
Marinoni M, Boscarol G, Bertoni E, Casciana ML, Tucci F, Capolsini I,
Notarangelo LD, Giordano P, Ramenghi U, Colombatti R. Monitoring oral
iron therapy in children with iron deficiency anemia: an observational,
prospective, multicenter study of AIEOP patients
(AssociazioneItalianaEmato-OncologiaPediatrica). Ann Hematol.
2020;99(3):413-420. https://doi.org/10.1007/s00277-020-03906-w
- Cancelo-Hidalgo
MJ, Castelo-Branco C, Palacios S, Haya -Palazuelos J, Ciria-Recasens M,
Manasanch J, Pérez-Edo L. Tolerability of different oral iron
supplements: a systematic review. Curr Med Res Opin. 2013;29:291-303. https://doi.org/10.1185/03007995.2012.761599
- Zimmermann
MB, Chassard C, Rohner F, N'goran EK, Nindjin C, Dostal A, Utzinger J,
Ghattas H, Lacroix C, Hurrell RF. The effects of iron fortification on
the gut microbiota in African children: a randomized controlled trial
in Cote d'Ivoire. Am J Clin Nutr. 2010;92(6):1406-15. https://doi.org/10.3945/ajcn.110.004564
- Røseth
AG, Fagerhol MK, Aadland E, Schjønsby H. Assessment of the neutrophil
dominating protein calprotectin in feces. A methodologic study. Scand J
Gastroenterol. 1992;27(9):793-8. https://doi.org/10.3109/00365529209011186
- Jukic
A, Bakiri L, Wagner EF, Tilg H, Adolph TE. Calprotectin: from biomarker
to biological function. Gut. 2021;70(10):1978-1988. https://doi.org/10.1136/gutjnl-2021-324855
- Ayling RM, Kok K. Fecal Calprotectin. Adv Clin Chem. 2018;87:161-190. https://doi.org/10.1016/bs.acc.2018.07.005
- Tibble
JA, Sigthorsson G, Foster R, Scott D, Fagerhol MK, Roseth A, Bjarnason
I. High prevalence of NSAID enteropathy as shown by a simple faecal
test. Gut. 1999;45(3):362-6. https://doi.org/10.1136/gut.45.3.362